All published articles of this journal are available on ScienceDirect.
Genetic Polymorphisms and Pesticide-Induced DNA Damage: A Review
Abstract
The drastic increase in pesticide applications makes human exposure inevitable either through environment or occupation. Pesticide toxicity causes many adverse health effects through a number of pathways leading to DNA damage, mutations and cancers. Nevertheless, there is heterogeneity in the degree of toxicity among individuals due to the influence of genetic polymorphisms on xenobiotic metabolizing enzymes (XMEs) that modulate the biological process. Thus, study of the most common polymorphic genes coding for the enzymes involved in pesticide metabolism (such as cytochrome P450, Glutathione S-transferases, N-acetyltransferase and paraoxonase) may help determine individual’s susceptibility to pesticide toxicity. In this review, we give an overview of some recent developments in the field of genetic polymorphism and pesticide-related DNA damage, including probable biomarkers that may uncover genome susceptibility to pesticide toxicity. We have tried to create a connection between DNA polymorphism and cancer onslaught globally. It is envisaged that knowledge on this line would improve our understanding of facilitating the association between genotype and phenotype in cancer biology.
1. INTRODUCTION
Pesticides are toxic chemicals that are widely used to kill insects and plant pathogens in agriculture. However, they are also used in several other processes such as food storage, sprays, and environmental activities like eradication of weeds and unwanted aquatic plants. Almost one-third of world’s crop production has been secured with pesticide application [1]. Furthermore, Pesticides’ application still remains the universally accepted way of boosting agricultural yields and control of pests. Pesticides constitute heterogeneous synthetic chemicals that humans inevitably encounter everyday through occupational use or environmental contamination [2]. Environmental contamination of pesticides poses a great hazard to human health. An estimated 2 million tonnes of pesticides are being used each year, with China being the largest consumer followed by the United States. India is among the 10 most widely utilized countries for pesticides and global consumption is estimated at up to 3.5 million tonnes by 2020 [3-5]. Bulk of these pesticide chemicals linger in the environment for a long time and their residual products (either through biological or physical degradation) get into the food chain through groundwater or air. These organic chemicals have deleterious effects on human health, ecosystem and wildlife [6]. When the ecosystem is affected, a large number of species and microbiotypes are affected. Therefore, humans are inevitably exposed to pesticides through the environment, having detrimental consequences on their health [7]. Environmental exposure to pesticides can lead to the development of different forms of cancer in humans [8]. On the other hand, occupational exposure to pesticides has been a concern, in particular with regard to chronic low-dose exposure due to non-compliance with safety measures or self-poisoning due to incorrect handling of pesticides [9]. Occupational exposure occurs throughout the pesticide-related activities ranging from formulations and mixing with solvents to applications such as spraying. These active ingredients of various chemicals together with their inert substances have long lasting cumulative effects on human health [10, 11]. People having occupational exposure to pesticides include company workers and those involved in transport and sales of such compounds, users such as farmers, gardeners, floriculturists and green house workers. Chronic exposure to pesticides is associated with several human diseases related to immune systems, reproductive systems, nervous system, respiratory and cardiovascular systems [12]. Occupational exposure to pesticides has been shown to be associated with risk of many cancers including hematopoietic ones [13].
Pesticides are often present as complex mixtures, which makes them more dangerous to health due to synergistic effects of different chemical constituents. Almost 900 pesticides active ingredients in the US are currently in the market through some 20,700 pesticide products [14, 15]. The major pesticide groups commonly used today are organophosphates (OP), carbamates, organochlorines, pyrethroids and Neonicotinoids. A number of studies have shown these pesticides to be genotoxic agents due to their ability to cause homeostatic imbalance and alteration of cellular components that collectively lead to chromosomal alterations and DNA damage (Fig. 1) [2, 16, 17]. This DNA damage is the underlying cause of mutation that ultimately leads to cancer [18, 19]. Since DNA damage is massive, the repair mechanism often fails to restore the damaged DNA. One of the recently hypothesized mechanisms through which pesticides cause DNA damage is oxidative stress. Oxidative stress often results from the disturbance of the balance between free radicals (such as hydrogen peroxide, superoxide anion, hydroxyl radical and singlet oxygen) generated by the pesticides and antioxidants. Under physiological conditions, antioxidants neutralize the free radicals to avert the occurrence of oxidative stress. However, pesticide imbalances can increase steady-state Reactive Oxygen Species (ROS) levels, stimulate ROS-induced cellular alteration that affects core homeostatic and regulatory processes, or overwhelm antioxidant defenses that collectively result in the production of oxidative stress [16, 20, 21]. ROS, by virtue of their large amount and high reactive nature, interact with DNA, thereby causing genotoxicity and mutations [22-24]. Oxidative stress has always been found to disturb cell signaling pathways, since ROS constitute the most important messengers in redox signaling [12]. In lieu of that, oxidative stress causes several diseases including cancers, neurodegenerative disorders, immunodeficiency syndromes, diabetes and respiratory disorders [25]. In addition to oxidative stress, pesticides have epigenetic modification tendencies, they are shown to regulate gene expression via DNA methylation and microRNAs (miRNA) de-regulation [26], and miRNA de-regulation is associated with the process of tumorigenesis [27]. Consequently, various in silico studies have been conducted to identify the specific miRNA or methylation hotspots related to the presence of diseases or for potential use as biomarker of exposure to hazardous substances [28-30]. Furthermore, pesticides have been linked to several cancers such as Hodgkin’s and non-Hodgkin’s lymphoma [31], leukemia [32, 33], multiple myeloma [34], lung cancers [11], as well as reproductive dysfunctions and congenital abnormalities [16].
DNA damage caused by pesticides can be reversed by cell’s repair system to a large extent or removed by apoptosis. However, the genotoxicity over the years may lead to further complications such as carcinogenesis, neurological and reproductive processes [35]. Despite the various mechanisms mentioned above, biological effects of pesticides seem to be modulated mainly by the occurrence of genetic polymorphisms. To be specific, individual’s genotype determines his/her susceptibility to DNA damage and cancer. Genetic polymorphisms in genes coding for DNA repairing enzymes and Xenobiotic Metabolizing Enzymes (XMEs) such as Cytochrome P450 (CYPs), Glutathione S-Transferases (GSTs), N-acetyltransferase 2 (NAT2), and paraoxonase 1(PON1) account for inter-individual differences in the ability to metabolize foreign substances (including genotoxic agents such as pesticides) and hence, leads to decrease or increase in one’s susceptibility to DNA damage, mutations and cancers [26]. Inheritance of certain allelic variants of genes coding for XMEs may lead to modulation of DNA damage. Some people may be more vulnerable than others to DNA damage caused by pesticides. This susceptibility can be examined by studying the most common polymorphisms of CYPs, GSTs, NAT2, and PON1 [26]. Additionally, exploring this human genetic variation could go a long way in saving the vulnerable group, because some disease-susceptible alleles occur more frequently among people of certain geographic areas. Unfortunately, fewer studies have examined the connection between genetic polymorphisms in XME, DNA damage and cancer [36], especially in pesticide-exposed populations. Even with that, several inconsistencies in the reports often make valid conclusions very challenging. However, certain degree of consistency has been observed in the recent reports linking genetic polymorphism of XME with risks of DNA damage and cancers, especially among individuals of the same ethnic or geographical locations [37-39].Therefore, the main objective of this review is to analyze the recent advancements (over the last decade) in the association between genetic polymorphism and pesticide-induced DNA damage.
2. DNA DAMAGE BY PESTICIDE
Pesticides, owing to their different chemical structure and compositions, have different modes of actions by which they eradicate the unwanted organisms. Some pesticides have anti-cholinesterase activity (such as OP), while some herbicides inhibit amino acid biosynthesis and photosynthesis, various fungicides hinder mitochondrial respiration while others hamper lipid biosynthesis and disrupt cell membrane and a lot of insecticides target multiple sites [40]. Apart from their distinct mechanism of actions, vast majority of these pesticides have been shown to induce DNA damage in humans through oxidative stress via different routes [40-42]. The pesticides induce oxidative DNA damage through generation of ROS via several metabolic processes. ROS are normal products of metabolic activities of the cell that function in signal transduction and immune system, they are counterbalanced by antioxidants in the body. However, this balance is perturbed by the pesticides through various mechanisms leading to the production of an overwhelming number of ROS that exert genotoxicity by binding to the DNA [43]. Furthermore, ROS attack and oxidize biomolecules- nucleic acids, lipids, proteins along with cellular components - thereby causing their fragmentations.
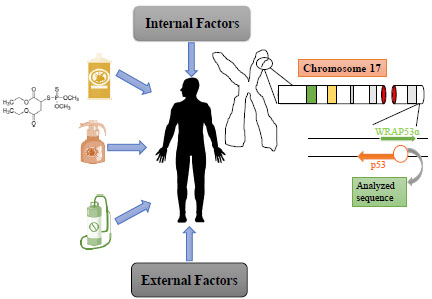
Organochlorine, methyl parathione, malathione and chlorpyrifos (the most commonly used pesticides), have been reported to cause oxidative stress by increasing ROS production and inhibiting cell antioxidant-associated enzymes or their biosynthesis [41, 44]. Normally, cells response to high ROS production by complexing DNA with histone for protection, minimizing respiratory processes in mitochondria and neutralizing excess ROS with superoxide dismutase and other anti-oxidant enzymes [45]. However, some pesticides tamper with the electron transport chains of mitochondria and endoplasmic reticulum thereby increasing the generation of ROS. Large amount of ROS can also be produced as byproducts of detoxification for various constituent chemicals of the pesticides. ROS and free radicals constitute the major contributors of human chronic diseases [43].
Free radicals and ROS bind covalently to form DNA adduct (Fig. 2). The DNA adducts can lead to mutations and ultimately carcinogenesis [46]. ROS binding to DNA can also result in Single-Stranded Breaks (SSB) which might later be converted to Double-Stranded Breaks (DSB). The DNA damage triggers events such as arrest of genome replication, transcription as well as induction of DNA repair. Moreover, DNA damage leads to generation of products from sugar breakdown such as 2-deoxypentonic acid lactone, erythrose, base-free sites and modified bases such as thymine glycol, 5,6-dihydroxycytosine, 8-hydroxyguanine, etc, which collectively leads to nucleic acid loss [46-48]. DNA repair system consists of enzymes capable of recognizing and repairing this damage, because efficient repair is essential to the cell survival and optimal function (Fig. 2). The efficacy of DNA repair hinges on the amount and duration of the damage [44]. Small DNA lesions, such as single base damage, are simply reversed in an error-free way or corrected via base excision repair (BER) pathway, while Nucleotide Excision Repair (NER) handles certain bulky lesions [49]. Persistent damage coupled with inefficient repair could result in mutations, chromosomal alterations and ultimately cancers. Therefore, ROS is associated with various types of cancers including ovarian cancers [50]. In addition, repeated ROS secretion produces an inflammatory micro-environment that promotes the transformation of ovarian cells into malignant cells in women [51].
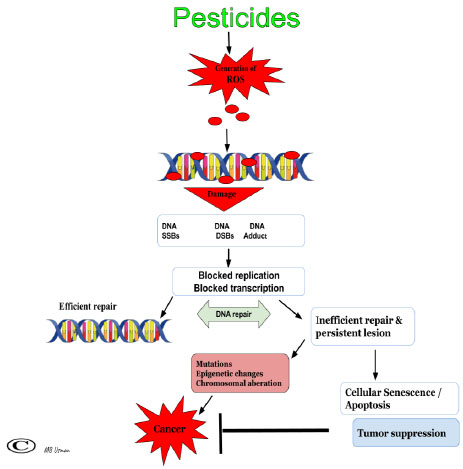
3. GENETIC POLYMORPHISM
Genetic polymorphism co-exists between two or more forms of allele at the gene locus with a frequency of approximately 1% or more in a given population, with the least common allele [52, 53]. This genetic variation emerges from inheritance of distinct DNA sequences and will result in different responses to substances among individuals or members of the same species [54]. Polymorphism in DNA repairing, and xenobiotic metabolizing genes often leads to altered activities of their corresponding enzymes. Certain allelic variants are associated with increased/decreased gene expressions changing the coding enzymes with fast or slow detoxification (metabolism) effect on endogenous and exogenous substances (like pesticides) (Fig. 3). Slow or inefficient DNA repair and detoxification can lead to accumulation of toxic substances, DNA damages and carcinogenesis. On the other hand, some mutant alleles can protect individuals from harmful effects of xenobiotics or make them more susceptible [26].
Genetic polymorphism in XMEs is a well-established phenomenon having a modulatory role on several mechanisms including DNA damage and cancers [52, 55]. Hence, our current focus is to uncover genetic variabilities and their impacts on gene expression and responses to various substances. It is envisaged that such allelic variation associated with the risks may be used as biomarkers for prognostic purposes.
3.1. Cytochrome P450s (CYPs)
CYPs are gene family that encodes enzymes (hemoproteins) capable of catalyzing phase I xenobiotic metabolism. CYPs are the primary enzymes involved in phase I biotransformation of xenobiotic substances (like pesticides) through oxidative process [56]. Various pesticides such as organophosphates are encountered through environmental or occupational exposures. These are converted into their bioactive forms (more toxic or carcinogenic) which facilitate their biological action, then into non-toxic forms to enhance their detoxification and excretion [26, 57].
CYPs are divided into families and subfamilies in accordance with their sequence. In humans, they are organized into 18 families with 44 subfamilies, the most important isoenzymes concentrated in human liver are CYP1A2, CYP2A6, CYP2B6, CYP2C9, CYP2C19, CYP2D6, and CYP3A4 which has the largest share of liver CYP proteins and metabolize about 50% of xenobiotics [58, 59]. Each of these subfamilies have several allelic variants. The major allele of CYP3A4 is CYP3A4*1B. Another important member of CYP superfamily is CYP2C9, which constitutes about 20% of hepatic CYP proteins, it has three alleles viz. CYP2C9*1, CYP2C9*2 and CYP2C9*3 [60, 61]. CYP2E1 and CYP1A1 are other important members of CYP family, known for their involvement in metabolism of several carcinogens. Genetic polymorphisms of CYPs grossly impact their metabolizing ability. Study of these polymorphisms may help in identifying biomarkers of susceptibility [62].
3.2. Glutathione S-Transferases (GSTs)
Human cytosolic GSTs consist of supergene family that encodes enzymes responsible for phase II detoxification of xenobiotics, through conjugation of various substrates having electrophilic moieties to Glutathione (GSH) [63]. GST enzymes catalyze the reaction of GSH with activated xenobiotic metabolites (such as pesticides and drugs) for detoxification and elimination [63]. Fukami & Shishido [64] first demonstrated the role of GST in metabolism of OP.
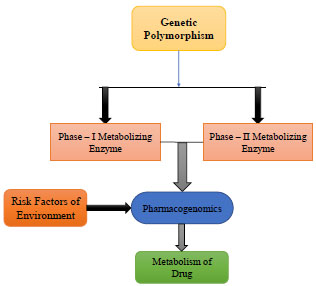
GSTs based on their sequence similarity, immune activity, and substrate specificity are categorized into seven classes namely Alpha (GSTA), mu (GSTM), pi (GSTP), theta (GSTT), kappa, and omega. These include GSTM1, GSTT1 and GSTP1 as the main enzymes for their functions in various exogenous and endogenous metabolisms. In addition, polymorphisms in their genes were found [39]. The presence of the polymorphic alleles accounts for the differences in expression levels and metabolic activities of the enzymes. Polymorphism of GST genes hampers metabolic elimination of toxic compounds and carcinogens, therefore, will lead to increased susceptibility to DNA damage and cancer [65, 66].
3.3. N-Acetyltransferases (NATs)
NAT is another gene that encodes enzymes involved in metabolism of various environmental toxins and drugs [67]. NATs catalyze the reactions of both activation (O-acetylation) and detoxification (N-acetylation) of arylamine and hydrazine groups of drugs and carcinogens [67, 68]. Humans express NAT1 and NAT2 genes, however, the latter is better known. Polymorphism of NAT2 gene in human leads to coding of enzymes with variable acetylation activities, showing slow, intermediate and rapid acetylation. Possession of slow or rapid acetylator genotypes is associated with higher risk of several diseases and cancers [67, 69, 70].
3.4. Paraoxonase 1 (PON1)
PON1 gene family codes for another enzyme involved in metabolism of lipid and organophosphate [71]. PON1 is a calcium-dependent enzyme belonging to three-gene family of paraoxonase (PON1, PON2, and PON3) that functions as esterase and lactonase [72]. Human serum PON1 is specifically involved in hydrolysis of lactones including Oxon metabolite of OPs pesticide [57, 73]. The two key coding zones involved in PON1 gene polymorphisms are: the PON1 192Q / R (responsible for the substrate-specific catalytic activity of the PON1) and the PON1 55L / M (related to enzyme serum concentration) [74]. Numerous researches concentrate on Q192R and L55 M polymorphisms. The 192R variant is more common in the studied populations, with a frequency of 25–64%, whereas the 55 M allele is less common in 5–40% of individuals [75]. Polymorphism in the isoforms of PON1 results in low or high activity of the enzyme leading to an increased activation or decreased detoxification of the toxic compounds. Individuals with higher PON1 activity are more likely to detoxify organophosphate [76], while individuals with lower PON1 activity are more vulnerable to parathionic intoxication [14]. The increased risk of lung cancer [77] and breast cancer [78] was also associated with PON1 polymorphism.
3.5. Genetic Polymorphism as Biomarker of Susceptibility
Polymorphism has been identified in almost all XMEs; several allelic variants have been implicated with various adverse health conditions or susceptibility to them. Susceptibility to undesired effects of toxic chemicals could, therefore, emerge from genetic or acquired traits, which results in metabolic instability and variability among individuals [37]. The metabolic variability stems from differences in the efficiency of the enzymes involved due to their polymorphic nature. Therefore, some alleles could be indicators (biomarkers) of susceptibility (Fig. 4). Humans exposed to pesticides who are also the carriers of this susceptibility-associated, metabolic and DNA genotypes that are likely to have higher risk of DNA damage [37, 79]. Several studies explored the possible use of certain polymorphic alleles as biomarkers of susceptibility to various disease conditions [37, 72, 78-82]. Biomarkers of susceptibility are closely related to that of early biological effect and exposure; they show higher vulnerability of individual to the diseases including cancers [82]. Various studies that investigated the role of specific polymorphisms of CYP2E1, GSTM1, GSTT1, GSTP1, PON1 and NAT2 in pesticide-exposed populations could be used in deducing potential biomarkers of susceptibility.
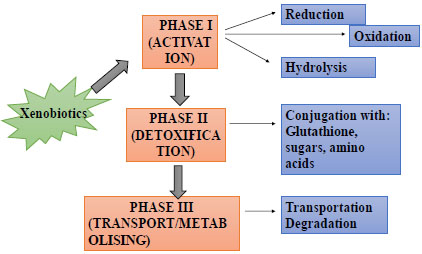
Research conducted by Sharma et al. [37], in India found that organochlorine-exposed individuals null deletions GSTT1 or GSTM1 deletions are more vulnerable to epithelial ovarian cancer. Furthermore, another recent study in India showed that pesticide-exposed agricultural workers with GSTT1 or GSTM1 null genotypes are more vulnerable to DNA damage, cancers and infertility [79]. In addition, Singh et al. [39], investigated the effect of GSTM1, GSTT1 and NAT2 polymorphisms on DNA damage and found that OP-exposed GSTM1 null genotype workers had higher DNA damage than their positive GSTM1 counterparts. Similarly, the workers with concomitant deletion of GSTM1 and GSTT1 genes have significantly greater DNA damage than those with the two intact genes. This result is in conformity with the one obtained on pesticide-exposed workers by Abhishek et al. [83], in Punjab (India); agricultural workers with GSTT1 gene deletions and those with simultaneous deletions of GSTT1 and GSTM1 are more susceptible to DNA damage. Even though there are minor differences between the findings, most of them showed increased susceptibility to DNA damage among the pesticide-exposed population with the presence of GSTT1 and / M1 null genotypes. This indicates the potentialities of the genotypes as biomarkers of susceptibility.
Some literature also investigated the polymorphism of CYP2E1, due to its involvement in metabolism of various endogenous and exogenous substrates and activation of toxic compounds and procarcinogenes [84]. Godoy et al. [85], reported an increased DNA damage together with higher number of heterozygotes in CYP2E1 gene among pesticide-exposed soybean farmers in Brazil, although no such association with genetic polymorphism was reported., Another study in Brazil showed that alcoholic individuals with heterozygous polymorphisms in the promoter region of CYP2E1 have higher DNA damage than those with homozygous non-mutated alleles [86]. Moreover, Kvitko et al. [87], observed high DNA damage among pesticide-exposed vineyard workers, they suggested that susceptibility to the DNA damage is associated with polymorphisms of CYP2E1, GSTM1 and GSTT1. Sameer et al. [88], found that CYP2E1 RsaI c2/c2 and CYP2E1 98-bp heterozygous i/I genotype are associated with higher risk of colorectal cancer and suggested that susceptibility to the cancer is related to the CYP2E1 polymorphisms. Therefore, CYP2E1 (heterozygous genotypes) could be a biomarker of susceptibility to DNA damage or cancer in pesticide-exposed populations.
Some researchers explored the polymorphisms of PON1 gene. Richard et al. [76], demonstrated the relationship between PON1 and cholinesterase activities using serum of pesticide-poisoned individuals and concluded that efficient detoxification of pesticide poisoning is more in individuals with high PON1 activity. Similarly, Kale [89] also showed that PON1 activity is significantly low in patients with OP poisoning, which indicate its suitability as a potent biochemical marker for prognosis and diagnosis of OP-based poisoning. For specific polymorphisms, You et al. [90], shown that Caucasians with PON1 192Q and 55LL polymorphic genotypes are more vulnerable to OP toxicity; however, similar phenomenon has not been observed among Asian population. Another meta-analysis shows that East Asians with PON1 Q192R and L55M polymorphisms are at higher risk of breast cancers, although the research outcomes are inconsistent [91]. Zayed et al. [92], suggested that PON1 Q192R polymorphism modulates patient’s response to acute OP intoxication. Additionally, Gómez-Martín et al. [74], in their study found linkage disequilibrium between the three PON1 polymorphisms analyzed (PON1 Q192R,PON1 L55M, and PON1 -108C/T), they concluded that the Spanish children living near agricultural area with adverse genotype combination of PON1 55MM/-108TT are more prone to OP toxicity. Therefore, determining PON1 status, which includes 192Q/R polymorphism and PON1 levels may help in ascertaining individual’s susceptibility to OP poisoning or cancer [93].
4. ASSOCIATIONS BETWEEN POLYMORPHISM OF XME AND DNA DAMAGE
In the past few years, the influence of XME polymorphisms in DNA damage has been given more importance [39, 94, 95]. The emphasis is on identifying the interaction between polymorphic gene and many pesticides in the gene-environment. It is believed that polymorphic XMEs modulate pesticide-induced DNA damage through gene-environment interactions which may lead to adverse health effects [37, 38, 39, 85, 96]. Therefore, combination of pesticide exposure and polymorphisms greatly modifies individual’s susceptibility to several diseases [26]. The susceptibility statuses of various polymorphic genes/ genotypes/ alleles to DNA damage/cancers are shown in the Table 1 and graphical representation in Fig. (5).
OPs are the most widely investigated pesticides (Table 1) and (Fig. 6A) owing to their commonness and commercial usage at both local and industrial scale. Although environmental exposure to pesticides has lethal effect, most of the studies pertinent to the pesticide’s toxicity focus on occupational exposures (Fig. 6B).
Recent studies investigated the polymorphisms of GSTs in risk determination among pesticide-exposed population. Sharma et al. [37], examined the relation of exposure to organochlorine pesticide and GSTM1, GSTT1 and CYP1A1 polymorphism in epithelial ovarian (EOC) cancer pathogenesis.100 Indian people with EOC cases and occupational exposure to organochlorine pesticides are participants. The result showed higher frequency of null deletions of GSTM1/T1 among EOC cases than in the 100 healthy (control) subjects. It is observed that polymorphism in the XME has modifier effect on the individual by predisposition to organochlorine pesticide, thereby putting him/her at higher risk. The authors concluded by proposing gene-environment interaction in the etiology of EOC. Another research conducted on 250 agricultural workers with occupational exposure to pesticide mixture in Punjab India associated GSTT1/M1 null genotypes with increased risk of DNA damage, cancer and others serious conditions, the authors suggested modulatory role of GSTT1 and GSTM1 in the pesticide-exposed workers [79]. Furthermore, a different study not mentioned in the Table 1 also emphasized the modulatory role of GSTM1 null genotype. Fortes et al. [52], explored the influence of GSTM1 polymorphism in altering the association of indoor pesticide exposure to cutaneous melanoma. The study involved 177 Italians with melanoma cases and indoor pesticide exposure. Fortes and colleagues observed increased risk of cutaneous melanoma among individuals with GSTM1 null genotypes who are exposed to indoor pesticides (≥ 2times / year) compared to GSTM1 active individuals with low exposure (< 2 times / year). Therefore, they suggested GSTM1 null genotype as risk modifier for cutaneous melanoma. This is similar to the finding of Singh et al. [102], who conducted their study on 115 Indians occupationally exposed to OPs. The GSTM1 null genotype was found to influence DNA damage in the exposed group more than the unexposed ones. Additionally, a case-control study investigated “gene-environment” interaction between polymorphism of GSTM1 and GSTT1 genes and organochlorine pesticides in the pathogenesis of Urinary Bladder Cancer (UBC). The study was conducted on 50 Indians with UBC and organochlorine exposure. The outcome showed significant increase in frequency of GSTM1/T1 null genotypes among UBC cases. Thus, individuals with GSTT1/M1 null genotypes are at higher risk of UBC in the course of their routine encounter with or exposure to organochlorine pesticides [38]. Moreover, Singh et al. [39], reported that workers with GSTM1 null genotypes and those with concomitant null deletions of GSTM1 and GSTT1 are more susceptible to DNA damage than those with both the genes who are equally exposed to OP pesticides. Abhishek et al. [83], also confirmed the same outcomes when GSTM1 and GSTT1 are simultaneously deleted. However, they found GSTT1 null genotypes (instead of GSTM1 null genotype in the previous study) to be associated with increased risk of DNA damage.
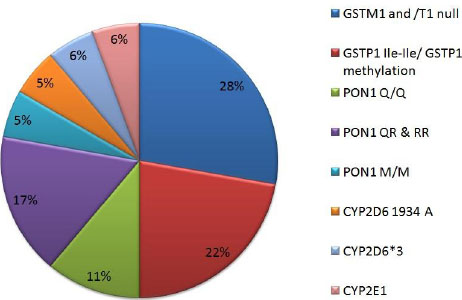
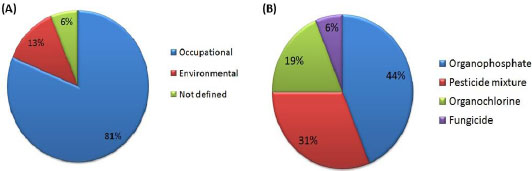
Study subjects/exposed/control | Pesticide | Exposure | Polymorphic gene/genotype/allele | Susceptibility to DNA damage/cancers | Reference |
---|---|---|---|---|---|
90/99 | Organochlorine | Environmental | GSTP1 rs1695 (Ile/Val+Val/Val) GSTP1 methylation GSTM1 & T1 deletions GSTP1 (Ile/Ile) & unmethylated |
Significant (p<0.05) Significant(p<0.001) Not significant Not significant |
[95] |
100/100 | Organochlorine | Environmental | GSTM1/T1 null GSTM1/T1 positive |
Significant(p=0.018) Not significant |
[37] |
74/89 | Pesticide mixture | Occupational | CYP2E1 | Not significant | [85] |
121/121 | Pesticide mixture | Occupational | PON1Q/Q (Gln/Gln) | Significant(p=0.013) | [96] |
250/263 | Pesticide mixture | Occupational | GSTM1/T1 Null |
Significant(p<0.0008) | [79] |
51/50 | Pesticide mixture | Occupational | GSTP1 Ile-Ile | Significant (p<0.005) | [97] |
30/29 | Organophosphate | Occupational | PON1 192RR CYP2D6 1934 A |
Significant (p=0.005) Significant (p=0.045) |
[98] |
54/54 | Organophosphate | Occupational | PON1 QR & RR | Significant (p<0.05) | [99] |
40/29 | Organophosphate | Occupational | PON1 192 RR CYP2D6 G1934A |
Significant (p=0.006) Not Significant |
[92] |
50/50 | Organochlorine | Occupational | GSTT1/M1 null | Significant (p<0.05) | [38] |
134/134 | Organophosphate | Occupational | GSTM1&T1 deletions GSTM1 & T1 Positive CYP2C9 *1/*2/*3 NAT2 slow acetylation |
Significant (p< 0.005) Not significant Not significant Not significant |
[39] |
108/65 | Pesticide mixture | Occupational | CYP2E1 | Significant (p<0.001) | [87] |
207/621 | Fungicide | Not defined | CYP1A1*2A | Not significant | [100] |
115/115 | Organophosphate | Occupational | PON1 Q/Q PON1 M/M (Met/Met) |
Significant (p<0.05) Significant (p<0.05) |
[101] |
115/115 | Organophosphate | Occupational | GSTM1 null GSTM1 positive GSTP1 Ile-Ile |
Significant (p=0.03) Not significant Significant (p=0.02) |
[102] |
150/134 | Organophosphate | Occupational | CYP 2D6*3 CYP 1A1 (m1 & m2) CYP3A5 (A44G0) CYP2C9 (*1,*2 & *3) CYP 2D6*4 |
Significant (p<0.05) Not significant Not significant Not significant Not significant |
[103] |
Although there is slight variation in the results which may be attributed to ethnic differences, high level of consistency is maintained particularly among Indian populations. Therefore, it may be commented that individuals with null deletions of GSTT1 and / or GSTM1 are more vulnerable to DNA damage and cancers (such as melanoma, urinary bladder cancer, epithelial ovarian cancers etc) than their positive counterparts when exposed to the same pesticides. The change in vulnerability can be due to polymorphisms in the XMEs which were thought to modulate the pesticide-induced cytogenetic changes through gene-environment interaction [95].
In order to further demonstrate the role of ethnic differences in results of research, a recent Chinese study found no relationship between gene deletions GSTT1 and GSTM1 and an increased risk of liver cancer. The study was conducted among 90 Chinese subjects with hepatocellular carcinoma and organochlorine exposure. The researchers however, noticed increased susceptibility to liver cancer among subjects with GSTP1 (Ile/Val+Val/Val) and GSTP1 promoter methylation than those with homozygous GSTP1 (Ile/Ile) and unmethylated [95]. Other studies related to GSTP1 polymorphisms are not consistent with the above one. For instance, Singh et al. [102], and Saad-Hussein et al. [97], both reported in their studies conducted in India and Egypt respectively that subjects with homozygous Ile-Ile GSTP1 genotypes are more susceptible to DNA damage than those with other alleles who are also exposed to the same pesticides. It may be observed that the results of Tian et al. [95], are not in line with Singh et al. [102], and Saad-Hussein et al. [97]. Although they are from different geographic locations, the latter results arrived at a common conclusion – suggesting the vulnerability of Ile-Ile GSTP1 genotypes to DNA damage. Therefore, more research needs to be done on GSTP1 polymorphism and its influence in altering pesticide toxicity, to determine the susceptibility status of various ethnic populations [95].
Other studies that investigated PON1 activity focused on polymorphisms of Q192R and L55M. Kahl et al. [96], studied the influence of polymorphisms of PON1, SOD (superoxide dismutase)2, OGG1, XRCC1 and XRCC4 genes in modulation of DNA damage among 121 tobacco farmers who are occupationally exposed to pesticide mixtures and nicotine in Brazil. There are higher rates of DNA damage in exposed individuals than in the control group, PON1 QQ (Gln / Gln) and SOD2 Val / Val genotypes have shown more association with damage and decreased antioxidant activity. Outcome of this research has projected the modulatory role of PON1 and SOD2 variants on DNA damage and its repair in the exposed subjects. Moreover, the outcome is consistent with that of Singh et al. [101], in India, which subsequently investigated 150 workers who were frequently exposed to OPs. In addition to the above finding, the authors also observed similar modulatory effects among individuals with PON1 MM (Met/Met) genotypes.
Contrary to the above results, a research conducted on 40 Egyptians who were occupationally exposed to OP showed that PON1 RR genotype and R allele carriers are more vulnerable to acute toxicity of OPs than other genotypes. The research objective was to determine the associations between genetic polymorphisms of PON1 Q192R, CYP2D6 G1934A and susceptibility to acute OP toxicity. The authors, however, did not observe any increase in susceptibility associated to CYP2D6 G1934A [92]. Furthermore, a more recent study also obtained the same outcome on cohort of 30 Egyptians chronically exposed to OPs [98]. The authors however, observed increased susceptibility to chronic OP toxicity among CYP2D6 1934 A allele carriers in addition to the abovementioned outcomes. Similar results obtained by Sunay et al. [99], revealed that Turkish subjects with occupational exposure to OPs who are also having PON1 192(R+)(QR + RR+ genotypes) genotypes are more susceptible to OP toxicity than 192 R(-) (QQ) genotype carriers.
Despite the discrepancies in the outcomes of various researches conducted on PON1 Q192R polymorphism, certain degree of consistency has been observed among cohorts of the same geographical or ethnic background. For instance, the outcomes of studies conducted on Egyptian and Turkish populations showed consistent results and indicated that individuals with PON1 RR genotypes and R alleles are at higher risk of acute or chronic OP poisoning [92, 98, 99]. On the other hand, PON1 QQ genotype carriers showed more sensitivity to OP poisoning among some Turkish and Indian studies. Inconsistency in results between studies may be related to a number of factors, along with ethnic variations and exposure to different OP compounds with distinguishable toxic effects [104]. Influence of ethnic variability on PON1 activity has further been demonstrated through the meta-analysis conducted by You et al. [90]. This meta-analysis included nine-case control studies that showed increased risk of OP toxicity associated with PON1 192Q and 55LL polymorphisms among Caucasians, but not in Asians. PON1 activities have also been shown to be affected by dietary habits, exposure to the environment (e.g. smoking) and certain drugs [93]. For CYP2D6 G1934A polymorphisms, inconsistent results have been reported. Singh et al. [103], found that Indian workers with CYP2D6*3 are more vulnerable to OP-induced DNA while Tawfik Khattab et al. [98], observed the vulnerability only in CYP2D6 1934 A allele carriers among Egyptian populations. Although ethnic differences may account for the inconsistency, both the two outcomes however, indicated the role of CYP2D6 G1934A polymorphisms in modulating OP toxicity. Therefore, both PON1 and CYP2D6 polymorphic genotypes can modulate DNA damage triggered by OPs possibly through gene-environment interactions [103].
CYP2E1 polymorphism reports also recorded some variability. For instance, Godoy et al. [85], did not find any significant association between CYP2E1 polymorphism and increased DNA damage, although they noticed higher level of DNA damage and more number of heterozygotes in CYP2E1 gene among the 74 soybean farmers with occupational exposure to pesticide mixture in Brazil. On the other hand, Kvitko et al. [87], observed increased susceptibility to DNA damage associated with CYP2E1 polymorphism among 108 vine-yard workers exposed to pesticide in Brazil. Although the two studies are from the same country, ethnic variability may account for the differences observed in the results. Further research is also needed to detect peculiarities of various ethnic groups and their susceptibility to various pesticides.
CONCLUSION
Genetic polymorphism in XME is thought to be a modifier of individual’s susceptibility to various diseases in the pesticide-exposed populations. Although the modulatory role of the polymorphic genes in DNA damage seemed to be the result of gene-environment interaction, extensive research on this line is prerequisites for better understanding of the basis of pesticide-induced DNA damage. Thus, identification of more high-risk genotypes and alleles would broaden our understanding. If this goal is achieved, that would facilitate the pesticide related screening of the masses and augment the development of better health policies. Moreover, future plans may include protective policies and measures to ensure that the vulnerable groups are protected from the unhealthy environmental and occupational exposures.
CONSENT FOR PUBLICATION
Not applicable.
FUNDING
None.
CONFLICT OF INTEREST
The authors declare no conflicts of interest, financial or otherwise.
ACKNOWLEDGEMENTS
The authors are grateful to Sharda University, N.N. Saikia College and Mahatma Gandhi Central University, India for support.