All published articles of this journal are available on ScienceDirect.
Immobilization of Cholesterol Oxidase: An Overview
Abstract
Background:
Cholesterol oxidases are bacterial oxidases widely used commercially for their application in the detection of cholesterol in blood serum, clinical or food samples. Additionally, these enzymes find potential applications as an insecticide, synthesis of anti-fungal antibiotics and a biocatalyst to transform a number of sterol and non-sterol compounds. However, the soluble form of cholesterol oxidases are found to be less stable when applied at higher temperatures, broader pH range, and incur higher costs. These disadvantages can be overcome by immobilization on carrier matrices.
Methods:
This review focuses on the immobilization of cholesterol oxidases on various macro/micro matrices as well as nanoparticles and their potential applications. Selection of appropriate support matrix in enzyme immobilization is of extreme importance. Recently, nanomaterials have been used as a matrix for immobilization of enzyme due to their large surface area and small size. The bio-compatible length scales and surface chemistry of nanoparticles provide reusability, stability and enhanced performance characteristics for the enzyme-nanoconjugates.
Conclusion:
In this review, immobilization of cholesterol oxidase on nanomaterials and other matrices are discussed. Immobilization on nanomatrices has been observed to increase the stability and activity of enzymes. This enhances the applicability of cholesterol oxidases for various industrial and clinical applications such as in biosensors.
1. INTRODUCTION
Enzymes are macromolecular biocatalysts, which accelerate biochemical reactions with high specificity and efficiency [1-3]. In recent years, enzymes have found immense applications in industrial bioprocesses, environmental remediation, biofuel production, pharmaceuticals and diagnostics [4]. However, many such applications of free enzymes face the drawback of poor stability, high cost and difficulty in reuse. The activity and stability of enzymes for the above applications have been improved by various methods [5]. Immobilization is one of the important techniques which have been exploited to improve enzyme characteristics. The macro/micro surfaces used in immobilization play significant role in enabling the use of enzymes in non-native applications such as in environmental remediation, biosensors, bioreactors and other applied biotechnology fields [6, 7]. The interaction of enzymes with material surfaces are of concern for many applications such as biosensors, biotransformations, in food industry, pharmaceutical transformations, imaging and drug delivery technologies. After immobilization on the matrix surfaces, the observed activity and specificity of the enzyme may undergo changes. Mostly, the enzyme properties are found to be enhanced in nature. However in some cases, enzymes lose their activity or undergo distortion in structure due to the physicochemical properties of the enzyme, nature of the material support and their mutual interactions [8, 9]. The multiple factors responsible for the limited functioning of the enzymes after attachment to the support include alteration of the native protein configuration, slower diffusion rates of the substrate towards the bulk-attached enzyme and steric hindrance [9-12]. Recently, many researchers have utilized Nanoparticles (NPs) as enzyme carriers for eliminating these negative effects of enzyme immobilization on macro/micro carriers.
Nanomaterials (particles with length scales < 100 nm), due to their high surface area to volume ratios possess unique characteristics that enhance biocatalysis. They also exhibit surface chemistry well-suited for bioconjugation, and length scales that integrate well with metabolic processes and gene expression [13]. These inherent capabilities make nanomaterials valuable for a wide variety of biotechnological applications including biosensing, drug delivery, bioremediation, biofuel production, antibacterial activity and disease diagnostics [14-24]. Nanomaterials have the ability to enhance the performance and activity of the immobilized enzyme [25, 26], as they influence the factors that determine the efficiency of biocatalyst, such as surface area to volume ratio, mass transfer resistance and effective enzyme loading [3, 27-35].
Cholesterol oxidase is a bacterial flavo-enzyme which finds great commercial value in the determination of cholesterol in food and clinical samples, as a catalyst for bioconversion of sterol compounds and as an insecticide [36]. The current review summarizes the different matrices and techniques for nanoimmobilisation, followed by discussion on some typical macro/micro and nanocarriers used for conjugating cholesterol oxidase and describes the applicability of such nano-preparations.
2. CHOLESTEROL OXIDASE
Cholesterol oxidase (EC 1.1.3.6) is a monomeric FAD-dependent oxido-reductase which catalyzes steroid substrates having a hydroxyl group at the 3-β position of the steroid ring. These enzymes are strictly of bacterial origin, and not found in plant or animal systems. First reported in Rhodococcus sp. [37, 38], cholesterol oxidase has been found to be produced by Pseudomonas sp. [39, 40], Burkholderia sp. [41], Mycobacterium sp. [42], Chromobacterium DS-1 [43] and Streptomyces sp. [44, 45]. among others. In fact, actinomycetes are the largest class of cholesterol oxidase producers. The enzyme exists both as membrane-bound and extra-cellular forms in bacteria. Based on their bonding to the cofactor FAD, cholesterol oxidases can be broadly divided into two classes:
Class-I cholesterol oxidases have non covalently-bound FAD, the covalent attachment found to consist of the imidazole ND1 atom of a histidine residue (His121) [46]. Rhodococcus and Brevibacterium cholesterol oxidase belong to this kind.
Class–II cholesterol oxidases have covalently bound FAD. The attachment to the protein, occurs through a bond linking the polypeptide chain to the 8-methyl group of the isoalloxazine moiety [47]. Cholesterol oxidase from Burkholderia and Chromobacterium belong to this kind.
2.1. Structure of Cholesterol Oxidase
The general structure of cholesterol oxidase consists of a substrate-binding domain and a FAD-binding domain, with the single protein chain meandering back and forth between the regions that provide the binding features for the cofactor and the substrate [47]. In most cholesterol oxidases, eight-stranded mixed beta-pleated sheet and six alpha-helices form the substrate binding domain. This domain is positioned over the isoalloxazine ring system of the FAD cofactor allowing oxidation of cholesterol and subsequent isomerisation to cholest-4-en-3-one [48]. The active site contains a hydrophobic pocket, sealed off from the outer environment by flexible loops [47].
In most flavin-containing enzymes, there exists a consensus sequence of repeating glycine residues (GXGXXG) followed by an Asp/Glu 20 residues further in the primary sequence, this motif forming the nucleotide binding domain [49]. The amino acid sequence, structure and redox properties vary a lot between the two different classes of enzymes. The noncovalent form of cholesterol oxidase exhibits a consensus sequence of glycines (G17-X-G19-X-G21-G⁄ A22) followed by a glutamate Glu40, indicating a nucleotide-binding fold. In the case of the covalently bound cholesterol oxidase, this consensus is notably absent [47]. In the non-covalent form, the FAD is bound to the protein by non-covalent interactions, which can be released only under denaturing conditions such as heating at 90°C.
2.2. Biological Functions of Cholesterol Oxidase
Cholesterol oxidase is found to play myriad roles in the bacterial metabolism. In cholesterol assimilating bacteria, it is involved in the first step of cholesterol metabolism, converting cholesterol to 4-cholesten-3-one [50, 51]. Bacteria such as Mycobacterium and Rhodococcus equi secrete cholesterol oxidase enzyme to damage the cell-membrane of the hosts, hence considered responsible for the pathogenticity of these bacteria [42, 52]. Additionally, product of the gene pimE coding for cholesterol oxidase in Streptomyces Natalensis, acts as a signaling protein for the production of pimaricin [53].
2.3. Applications of Cholesterol Oxidase
An important application of cholesterol oxidases is as a diagnostic tool to determine cholesterol levels in serum [54], HDL, LDL [55], on the cell membrane of erythrocytes [56], in human bile and in gall stones [57], in atherosclerotic diseases and other lipid disorders. In most cases, the enzyme is accompanied by cholesterol esterase, EC 3.1.1.13 (which frees esterified cholesterol present in blood serum) and peroxidase EC 1.11.1.7 (which reacts with hydrogen peroxide and a dye to give a colored end product) to aid in detection. This reaction forms the primary basis for most cholesterol biosensors having immobilized forms of cholesterol oxidase.
Cholesterol oxidase proves to be an effective biocatalyst for the transformation of cholesterol and other sterol compounds to form pharmaceutically important products such as 4-cholesten-3-one, androst-4-ene-3,17-dione and androsta-1,4-diene-3,17-dione [58]. It could also be used in the bioconversion of cholesterol into bile acids, where cholesterol oxidase is used to convert 3β, 7α-cholest-5-ene-3,7-diol to 7α-hydroxycholest-4-en-3-one [59]. Cholesterol oxidase from Rhodococcus erythropolis was employed for the preparative oxidation of cyclic allylic, bicyclic and tricyclic alcohols [60]. These enzyme mediated processes are eco-friendly, cost effective and operate under milder reaction conditions.
In the early 1990s, Monsanto Co. (St Louis, MO, USA) discovered a highly efficient insecticidal protein effective against the boll weevil (Anthonomus grandis Boheman) larvae and other lepidopterans [61]. This was later found to be cholesterol oxidase with an insecticidal activity at 50% concentration (LC50). When cholesterol oxidase is included in the diet of the larvae, it induces lysis in the midgut epithelium. However, adult boll weevils are insensitive to cholesterol oxidase. Transgenic leaf tissues expressing ChOx have also been found to exert insecticidal activity against boll weevil larvae [62].
Cholesterol oxidase provides an interesting target for bacterial infections from species such as Rhodococcus equi, which resides in the macrophages of hosts and acts as an opportunistic pathogen in immune-compromised individuals. Mycobacterium tuberculosis and Mycobacterium leprae have also been found to produce cholesterol oxidase [63]. The bacteria are found to induce membrane lysis facilitated by the induction of extracellular cholesterol oxidase, thus can be used to target the virulence [52].
3. IMMOBILIZATION OF ENZYMES
3.1. Immobilization Methods
Generally, immobilization of enzymes can be carried out by physical as well as chemical methods. In physical methods, the enzyme is bound to the matrix by weak interactions, while in chemical methods, covalent bond is formed between the matrix and the enzyme. Recently, the advances in organic chemistry and molecular biology for protein immobilization to support resulted in the development of some very potent, efficient and site-specific applications such as functional protein microarrays, biosensors, and continuous flow reactor systems [2, 30, 64-66]. Broadly, enzyme immobilization methods can be divided into three categories, direct binding to a support, entrapment and cross-linking as summarized in Fig. (1). In each case, researchers try to maintain the catalytic activity while achieving the expected technological advantages through immobilization protocols. However, the stress developed by the immobilization procedures may induce conformational changes and partial loss of the enzyme activity [67].
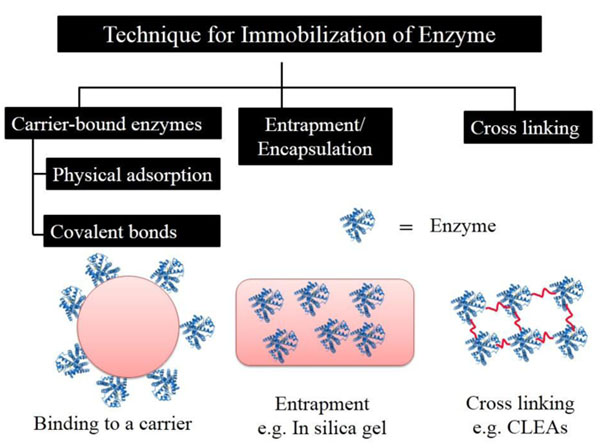
Binding to a carrier can occur through adsorption or covalent attachment processes [66]. The adsorption through the physical method generally involves multipoint protein adsorption between the protein molecule and binding sites on the immobilization surface through ionic and hydrogen bonding, van der Waals forces and hydrophobic interactions [68, 69]. In these methods, the enzyme is easily desorbed from the immobilization surfaces by fluctuations of pH and temperature [70]. A few advantages of the adsorption methods are that they are easy to carry out, no reagents are involved, minimum activation step is required, are comparatively cheap, and are less disruptive to protein structure than most chemical methods. Covalent coupling is the most frequently used approach to immobilization in which covalent bonds are formed between surface amino acids of the enzyme and the matrix. The advantages of the covalent bonding method include strong linkage of enzyme to the support (due to which no leakage or desorption occurs), a comparatively simple operation, availability of a variety of supports with different functional groups, and wide applicability. The disadvantages of the covalent bonding method include chemical modification of the enzyme leading to functional conformational loss and enzyme inactivation by a change in the conformation of the active site. This can be overcome through immobilization with the substrate or a competitive inhibitor of the enzyme.
Entrapment of enzymes involves the inclusion of the protein in a polymer matrix, such as silica sol–gel and polyacrylamide or artificial membranes like microcapsule and hollow fiber. However, the enzyme leakage cannot be entirely prevented due the weak nature of the physical bonds, therefore implicating additional covalent attachment. For efficient entrapment, the synthesis of the polymeric matrix must take place in the presence of the enzyme. Thermo reverse polymerization has been used to entrap enzymes in natural polymers such as agar, agarose and gelatin, while ionotropic gelation has been used for alginate and carrageenan matrices [71]. Additionally synthetic polymers like polyvinyl alcohol hydrogel [72], polyacrylamide [73] have also been explored for their application in enzyme entrapment. The advantages of the entrapment method are that it is fast, inexpensive, milder conditions are required, and there is less chance of conformational change in the enzyme. The disadvantages include leakage of the enzyme, pore diffusion limitation, and chance of microbial contamination. Immobilization of cholesterol oxidase by entrapment methods have been done by many researchers [74, 75].
Cross-linking of enzyme aggregates is obtained by binding of enzymes to each other by bi- or multifunctional reagents or ligands to prepare carrierless macroparticles [71]. Cross-linking is a simple immobilization process as it does not involve any support matrix. In this method, covalent bond is formed between the biocatalyst, because of which the biocatalyst may undergo conformational changes, resulting in activity loss. Glutaraldehyde is a bifunctional agent generally used for cross-linking. It has aldehyde groups at both ends to react with the free amino groups of enzymes. Thus, carrier-free immobilized enzymes, such as Cross-Linked Enzyme Crystals (CLECs), and Cross-Linked Enzyme Aggregates (CLEAs) are finding increasing interest in the industries [76, 77]. The advantage of the cross-linking method is that it is issued mostly as a means of stabilizing the adsorbed enzyme and also for preventing leakage. The disadvantage of this method is that it may cause significant change in the active site of the enzyme which may lead to a loss of activity.
The factors that determine the category of immobilization to be employed depend on the type of enzyme and the matrix used. Whenever a new immobilization protocol is developed, importance should be given to enzyme recovery percentage, selectivity, operational stability, and reduction in inhibition by the products or any other component of the media. The interaction between the matrix and the enzyme should be controlled and its catalytic properties maintained by proper orientation of the protein. Most importantly, use of toxic and highly unstable reagents during the immobilization processes should be kept to a minimum, making it eco-friendly as compared to other established technologies.
3.2. Choice of Support for Immobilization
Support material is one of the major components for the immobilization of enzymes. Thus, it should be possessed with some important characteristics like large surface area, suitable shape and particle size, high rigidity, hydrophilic character, permeability, insolubility, chemical, mechanical and thermal stability, resistance to microbial attack, and regenerability [78]. Support materials can be classified based on morphology (porous and nonporous support) and their chemical nature (organic and inorganic). Organic support materials are further classified as natural and synthetic. Natural organic supports include polysaccharides and proteins [79, 80]. The surface of most inorganic supports is mainly composed of oxide and hydroxyl groups, such as silanol group in glass, and provides a mild reactive surface for activation and protein binding [71, 81, 82].
Properties of the immobilized enzyme inevitably depend on the choice of the matrix. Nanomaterials possess the ideal size as immobilization matrices as they occupy lesser volume in bioreactors as compared to conventional matrices while providing high surface/volume ratio. Nanoscale materials also have additional advantage of low cost, rapid reaction, mild conversion conditions, robust activities, mobility, high loading, operational stability and minimum diffusional limitation [83]. Moreover, the small size and multifunctionality of nanoparticles (of metals such as Au, Fe, Zn and Ag) make them suitable to interact and operate at biomolecular levels finding applications in biosensors, tumor location analysis and drug delivery [84].
4. IMMOBILIZATION OF CHOLESTEROL OXIDASE
Cholesterol oxidases from bacterial sources have been immobilized to improve their enzymatic properties, reusability and operational stability. The current section summarises some of the established instances of cholesterol oxidase immobilization onto various macro/micro and nanocarriers.
4.1. Immobilization on Macro/ Micro Carriers
To improve its catalytic properties, various macro/micro carriers have been employed to immobilize cholesterol oxidase. Cholesterol oxidase from Rhodococcus sp. NCIM 2891, immobilized on chitosan beads was found to have increased stability in organic solvents, and was reusable upto 12 successive cycles of operation. This immobilized preparation was used for the biotransformation of cholesterol to 4-cholesten-3-one with around 88% millimolar yield [85]. Two important reports by the same group describe the co-immobilization of Cholesterol Oxidase (ChOx) with Horseradish Peroxidase (HRP) and Cholesterol Esterase (ChEt) respectively [74, 86]. While horseradish peroxidase aids in colorimetric detection of cholesterol, cholesterol esterase breaks down the esterified cholesterol present in blood serum into free cholesterol for easy detection. The co-immobilization was carried out on Tetraethyl Orthosilicate (TEOS) derived sol-gel films, by physisorption, physically entrapped sandwich and microencapsulation with ChOx/HRP while covalent modification was carried out for the preparation with ChOx/ChEt. The sol-gel/ChOx/ChEt complex reveal high thermal stability upto 55°C and detection limit of 12 mg dL−1 and sensitivity of 5.4×10−5 Abs. mg−1 dL−1 for cholesterol [74]. All the three techniques used for the immobilization of ChOx/HRP showed linearity for 2-10 mM cholesterol [86]. A similar co-immobilization of ChOx, ChEt and HRP was carried out on alkylamine glass beads and PVC [87, 88]. N-ethyl-N’-3-dimethylaminopropyl carbodiimide activated sepharose is another popular matrix for immobilization of cholesterol oxidase. The immobilized preparation retained 90% of activity at 4 and 9 pH, but did not possess commendable storage stability [89]. Conducting polymers such as thiophene-3-boronic acid and 3-aminophenyl boronic acid increase the interaction between the enzyme and the electrode and have been used to immobilize Brevibacterium sp. cholesterol oxidase for biosensing applications [90]. Cholesterol oxidase has also been conjugated to polymers such as electrochemically-polymerized polyaniline with Triton X-100 and functionalized poly(methyl methacrylate-co-glycidyl methacrylate) and poly(acrylamide-co-acrylicacid)/polyethyleneimine supports [91, 92].
4.2. Immobilization on Magnetic Nanoparticles
Even though a variety of matrices have been used to immobilize cholesterol oxidase, reports on magnetic nanoparticles have been scarce. Many such studies employ co-precipitation of magnetic Fe3O4 nanoparticles with other matrices. For application as a biosensor, nanoparticles synthesized by the co-precipitation of Fe2+ and Fe3+ and functionalization with poly(styrene-co-acrylic acid) have been used to conjugate cholesterol oxidase followed by deposition on the planar platinum polyaniline (PANi) modified electrode [93]. An attempt at the immobilization on magnetic fluorescent nanoparticles, was done through APTES mediated covalent coupling [94]. The maximal catalytic activity was obtained at pH 7.0 and 50 °C. The immobilized preparation retained 80% of its activity at 50ºC for 5h and it was reusable for 7 consecutive operations. Interestingly, the immobilized enzyme was optically sensitive to oxygen in solution, thus rendering it applicable for fibre optic based biosensors. A similar study on immobilization of cholesterol oxidase from various bacterial sources was carried out on APTES modified iron (II, III) oxide nanoparticles followed by glutaraldehyde crosslinking. These nanoconjugates increased the thermal and pH stability and were further used for the production of 4-cholesten-3-one and 4-cholesten-3,5-dione [95]. Cholesterol oxidase has also been attached to magnetic nanoparticles (Fe3O4) synthesized by thermal co-precipitation of Fe2+ and Fe3+ chlorides, through carbodiimide linkage. Such conjugation of cholesterol oxidase resulted in almost 98-100% binding with enhanced pH and temperature stability [96]. Functionalized silica-coated magnetic nanoparticles have also been used for cholesterol oxidase immobilization. Synthesis of maghemite (γ-Fe2O3) nanoparticles was done by thermal co-precipitation of iron ions in alkaline ammonia solution, followed by deposition of silica. Secondary functionalization was done using organosilane and cross-linking by glutaraldehyde [97]. Cholesterol oxidase immobilized on the silica-coated maghemite has been found to retain around 60% of its activity [98]. Cholesterol biosensors have also been developed by co-immobilizing cholesterol oxidase with Fe2O3 micro-pine shaped hierarchical structures with very high and reproducible sensitivity and low detection limit [99].
4.3. Immobilization on Metal Nanoparticles
Majority of the cholesterol oxidase biosensors comprise of gold as the electrode. Thus, gold (and other metal) nanoparticles aid in the deposition of cholesterol oxidase on the electrode surface. In one such report, gold electrode was modified with 1,6-hexanedithiol, followed by deposition of gold nanoparticles. The surface of the nanoparticles was functionalized with carboxyl groups using 11-mercaptoundecanoic acid and cholesterol oxidase was immobilized on the surface of the gold nanoparticle film by covalent bonding using the N-ethyl-N’-(3-dimethylaminopropyl carbodimide) and N-hydroxysuccinimide ligand chemistry [100]. A novel biosensor for quantitatively estimating cholesterol in pork liver and egg yolk samples was made by a glassy carbon electrode with nano-composite mixture consisting of Molybdenum Disulfide (MoS2) and gold nanoparticles (AuNPs), over which cholesterol oxidase was deposited [101]. Another interesting take on the cholesterol oxidase nanoimmobilization was the synthesis of aggregates of enzymes in the nanometer scales. The aggregates were achieved by cross-linking of cholesterol oxidase and cholesterol esterase with glutaraldehyde followed by deposition on Au electrode for manufacturing the biosensor. These ENPs retained 50% of initial activity during its regular use over a period of 60 days when stored at 4°C [102].
4.4. Immobilization on Other Nanoparticles
In addition to conventional nanomatrices, various novel materials have been explored for their capacity to immobilize cholesterol oxidase. Successful immobilization of cholesterol oxidase has been carried out on Mg2AlCO3 Hydrotalcite Layered Double Hydroxide nanomaterials for biosensor applications [103]. This group used extracts of Acacia salicina leaves in order to scavenge the free radicals such as H2O2 liberated during the enzymatic reaction. Polymers such as Poly-Ethylene Glycol (PEG), decorated polystyrene (PS) nanoparticles along with Congo red have also been used to immobilize cholesterol oxidase [104]. APTES modified and glutaraldehyde cross-linked silica nanoparticles were used to conjugate cholesterol oxidase, subsequently used as a biosensor with 200s response time and detection limit of 4.2 mg/dL [105].
Immobilization of enzyme to improve upon their biochemical properties, stability and reusability has been explored for a variety of industrial and clinically important enzymes. Apart from cholesterol oxidase, enzymes successfully conjugated onto macro/micro carriers as well as nanoparticles include cellulose [30, 106] keratinase [107, 108], lipase [109, 110] laccase [111, 112] and α-amylase [113, 114]. From the biosensing point of view, enzymes which has been immobilised apart from cholesterol oxidase include glucose oxidase [115], horseradish peroxidase [116], glucose dehydrogenase [117] and lactate dehydrogenase [118]. In fact, glucose oxidase forms one the largest class of oxidase enzymes immobilised for clinical applications.
5. APPLICATIONS OF IMMOBILIZED CHOLESTEROL OXIDASE
Immobilization of cholesterol oxidase renders it useful for varied industrial and medical applications. The majority of immobilized forms have been used as biosensors for the detection of cholesterol in food samples, blood serum and other clinical samples. Other applications include bioconversions for the production of steroid compounds. The general enzymatic cholesterol biosensors comprise of cholesterol esterase (which converts cholesterol esters present in blood to free cholesterol), cholesterol oxidase (which converts the free cholesterol to 4-cholesten-3-one) and horseradish peroxidase (it reacts with the liberated H2O2 to result in the colored compound which is detected). However, a limitation of this specific cholesterol detection scheme is the high cost of enzyme involved. Cholesterol oxidase based biosensors are considered superior as compared to traditional detection methods such as HPLC or GC, owing to their simpler working protocols, higher reusability, higher specificity and low cost.
Ordinary fabricated biosensors are limited by inadequate performance in terms of detection limit, sensitivity and stability. These challenges can be overcome by the use of nanomaterials, which provide catalytic, optical and electronic properties. Incorporating nanomaterials in biosensors enhance the surface chemistry, electroconductivity and compatible length scales for bioconjugation. Some of the nanomatrices used for the immobilization of cholesterol oxidase for biosensing applications are discussed here.
The physicochemical properties of cholesterol oxidase biosensors are generally studied through electrochemical impedance spectroscopy and cyclic voltammetry. In one report, the linear range of the biosensor made by electrodepositing cholesterol oxidase nanoparticles was 10-700 mg/dL for cholesterol. It optimally responded at pH 5.5 and 40°C within 5s when polarized at +0.25 V versus Ag/AgCl [101]. A gold based biosensor was created by covalently conjugating cholesterol oxidase to gold nanoparticles, which in turn were deposited on a gold electrode [100]. This biosensor exhibited a linear response to cholesterol in the range of 0.04-0.22mM with a detection limit of 34.6µM, apparent Km of 0.062mM with cholesterol and a high sensitivity of 9.02µA mM−1. Polymeric biosensors created by cholesterol oxidase immobilization onto Polystyrene/PEG/Congo red particles yielded linear response in the cholesterol concentration range of 100 mg dL−1 to 300 mg dL−1 [104].
Immobilization of cholesterol oxidase has proved to be a versatile technology for medical and food applications. For an enzyme like cholesterol oxidase with varied clinical applications, it becomes imperative that the substrate specificity is maintained after immobilization. However, the effect of immobilization on substrate specificity need to be studied further.
CONCLUSION
The science of immobilization of enzymes and other biological molecules on different supports is now well established and developed earlier. Selection of appropriate support matrix in enzyme immobilization is of extreme importance. Recently, nanomaterials have been used as a matrix for immobilization of enzyme due to their large surface area and small size. In this review, immobilization of cholesterol oxidase on nanomaterials and other matrices were discussed. Immobilization on nanomatrices has been observed to increase the stability and activity of enzymes. This enhances the applicability of cholesterol oxidases for various industrial and clinical applications such as in biosensors.
CONSENT FOR PUBLICATION
Not applicable.
CONFLICT OF INTEREST
The authors declare no conflict of interest, financial or otherwise.
ACKNOWLEDGEMENTS
Shubhrima Ghosh is grateful to the Indian Institute of Technology Delhi for Senior Research Fellowship. Razi Ahmad is thankful to DST-SERB [PDF/2016/000090] for providing national postdoctoral fellowship.