All published articles of this journal are available on ScienceDirect.
Application of CRISPR/Cas9 Genome Editing System to Reduce the Pre- and Post-Harvest Yield Losses in Cereals
Abstract
Cereals are an important source of food for millions of people across low-middle-income countries. Cereals are considered a staple food for poor people. The majority of the people are depending upon agricultural occupation. Agriculture provides a primary source of income for many farmers in low-middle-income countries. The pre- and post-harvest loss of crop yield affects farmers and is a major problem in achieving food security. Biotic and abiotic factors cause pre- and post-harvest loss of crop yield worldwide. It significantly affects the economic status of farmers as well as low-middle-income countries. Many advanced technologies are available for resolving the pre- and post-harvest loss of crop yield. The past few decades have seen remarkable progress in crop improvement. Especially high-throughput genome sequencing approaches contributed to advancement in the crop improvement. Genome-editing has also been considered a key tool for crop improvement. The clustered, regularly interspaced, short palindromic repeats (CRISPR)/CRISPR-associated protein 9 (Cas9) system has become a potent genome editing system for modifying key traits in cereal crops. CRISPR/Cas9 system offers new opportunities for addressing pre-and post-harvest constraints affecting cereal grain production and storage. In this review, we discuss the application of the CRISPR/Cas9 genome editing system to reduce pre-and post-harvest yield loss in cereal crops. It may promote the economic status of farmers and reduce food demand in the coming decades.
1. INTRODUCTION
Cereals are the edible grains and belong to the grass family, Poaceae (Gramineae) [1]. It includes rice, maize, wheat, rye, sorghum, barley, oats, triticale, millets, etc. Cereals are the most important food and nutritional crops in the world [2]. Cereal crops can be consumed in different ways, which are used to make many healthy dishes for humans [3, 4]. Major crops such as rice, maize, and wheat provide more than 30% of the food calories to 4.5 billion people in developing countries [5]. Other cereals and non-cereal crops are also important for human health [6-9]. They have a significant role in food security. Global food and nutritional security depend upon sustainable cereal crop production. The agricultural sector provides a primary source of income for farmers in developing countries. It depends upon the production and marketing of crops. The pre- and post-harvest loss of crop yield is a major issue in the current scenario [10]. This makes debt a liability for farmers and also it leads to food demand in the future. Both biotic and abiotic stresses influence the pre-and post-harvest loss of crop yield. For example, drought stress may occur at the panicle emergence or grain filling or grain maturation time of the crop plants, which severely affects the loss of yield. Many researchers have reported that the incidence of drought at the pre-harvesting stage has significantly reduced the grain yield [11-13]. The unexpected rainfall also causes the pre-harvest sprouting of seeds in the mother plants. It is one of the serious issues for pre-harvest loss of yield. Pre-harvest sprouting not only makes a serious economic issue for farmers but also leads to reducing crop yield and quality [14]. The insect and pests also significantly affect the pre-harvest loss of crop yield [15]. After post-harvest, correct storage facilities of the grain are important to prevent yield loss due to pest attack, mould spoilage, grain sprouting, etc [16]. It is very difficult to store cereal crops on a large scale due to a lack of storage facilities. Reducing pre- and post-harvest yield loss could be a sustainable solution to reduce the food demand [17]. Various approaches to improving the crop traits and storage facilities, adopting better agronomic practices, etc. may help to reduce the pre- and post-harvest loss of yield. These are the most important approaches to increasing agricultural productivity.
In past, chemical-based insecticides, pesticides, fungicides, and other chemicals were used for increasing the shelf life and decreasing the yield loss of the crops [17-19]. It may lead to many health issues in human beings due to chemical toxicity [20-22]. The consumption of these crops is not good for human health. For example, chemical fumigants such as ethylene dibromide, methyl bromide, and ethylene oxide are very risky to human health and the environment [22]. These chemicals are widely used to control insect infestations in crops. Developing an improved crop variety with an increased shelf life of the yield is an important approach for reducing the pre- and post-harvest loss of yield. It is safe for human health and avoids environmental risks. Many advanced genomic approaches are available now for resolving this issue. Genome sequencing technology helps to understand the genome organization of crops [23-26]. It helps to improve crop traits through molecular breeding and genome-editing approaches [27, 28]. Clustered, regularly interspaced, short palindromic repeats (CRISPR)/CRISPR-associated protein 9 (Cas9) is a key genome editing system and is widely used for altering plant traits [29-32]. CRISPR/Cas9 system is applied in many crop plants and improves their traits under various biotic and abiotic stresses [33-38]. Researchers have adapted the CRISPR/Cas9 system as an efficient genome-editing technique in targeting the gene of interest for crop plants. It may also help to improve crop traits and reduce the pre- and post-harvest loss of yield. In this review, we discuss the application of the CRISPR/Cas9 genome editing system to reduce pre-and post-harvest yield loss in cereal crops. This review will help researchers to understand the scope and application of the CRISPR/Cas9 genome editing system in crop improvement, which may help reduce food demand in the coming decades.
2. OVERVIEW OF POST- AND PRE-HARVEST YIELD LOSS IN CEREAL CROP
The economy of the developing country is mainly dependent on agriculture. Agriculture is the backbone of developing countries, and also it is a leading occupation for people. Cereals are major food crops for developing countries and are providing food security. Global cereal production is around 2788 million tons per year [39]. Cereal grain production is constrained by both biotic and abiotic stresses [40-42]. During the pre- and post-harvest loss of crop yield due to biotic and abiotic stresses, the economic value of the crops is reduced or makes them unsuitable for human consumption. It may significantly affect the overall cereal production worldwide. For example, more than 70% of the African (sub-Saharan region) population is directly involved in agriculture [43]. The post-harvest loss of crop yield is estimated to be between 20 and 40% in the African region [44]. The post-harvest losses are valued at around the US $1.6 billion per year. Such economic losses are a combination of those which occur on the field during harvesting time, in storage, during post-harvest processing, and during other sales and marketing activities. Pre-harvest sprouting is a major issue in decreasing cereal production worldwide [45]. It also severely affects the quality of cereal crops such as rice, maize, wheat, barley, oats, rye, etc [46-49]. Pre-harvest sprouting causes an annual economic loss of one billion dollars on a global scale [50]. Crop yield loss from insects and pests’ infestation before harvest and during storage time are serious problems in developing countries [17, 51]. It is estimated that 30-40% of stored grain is damaged annually by insects and pests [52]. Other various biotic and abiotic factors are also severely affecting the pre and post-harvest loss of crop yield [53-57]. It leads to a decrease in the economy of a developing country. Reducing pre- and post-harvest loss of crop yield is a major research area in the current scenario. Agriculture scientists need to adopt new techniques to reduce pre-and post-harvest loss. It may improve the economic status of farmers as well as developing countries.
3. FACTORS AFFECTING THE PRE- AND POST-HARVEST YIELD LOSS IN CEREAL CROP
Crop production and productivity are influenced by various agro-ecological topography. Proper agricultural practices are critical for reducing yield loss. The reduction of crop yield is influenced by many factors. These factors are categorized into three such as 1) technological (agricultural practices, storage facilities, transportation, and marketing, etc), 2) biotic/ biological (insect, pest, disease, etc.), 3) abiotic/ environmental (drought, moisture, soil fertility, etc.). Biotic and abiotic factors are a major problem in the pre-and post-harvest loss of agricultural products (Fig. 1). Significant yield losses from both biotic and abiotic stresses have been reported by many researchers [58-62]. For example, abiotic stresses such as temperature, drought, and low soil fertility, cause up to 82% annual loss of crops yield worldwide [63]. A global survey indicated that insects and pests cause yield losses of up to 30% in crops [64]. Haque et al. [31] reported that pre-harvest yield losses due to diseases can be up to 15% in major food crops. The pathogenic micro-organism causes more than 42% yield losses and reduces 15% of global food production [65]. There is a huge impact of individual biotic and abiotic constraints reducing the crop yield and quality, which may lead to food demand in the coming decades. The main reasons for the pre-and post-harvest loss are 1) delay of grain maturation, 2) unexpected rainfall, 3) grain shedding, 4) insect and pest attack during grain development, and 5) storage pest damage, etc. Effective agriculture practices are essential for reducing the loss of yield at the time of pre-and post-harvest stages.
In abiotic stress, moisture, temperature, and drought are the main factors affecting crop production, which is highly influenced by the pre- and post-harvest loss of grain yield. Pre-harvest sprouting has been recognized as one of the main factors that reduce the production and quality of crops [66]. It is due to the incidence of moisture contents before harvest. Pre-harvest sprouting issues have been reported in rice, maize, wheat, barley, etc [67-73]. The accumulation of high moisture content in the grains also leads to fungal infections [53, 74, 75]. This also reduces the quality of the crop and makes it unfit for human consumption. Optimum temperature is necessary for harvesting and storage. Proper temperature is very effective for maintaining crop quality. The drought stress mainly decreases the harvest index and delays the seed maturation of the crops [76]. The insect and pests are the main factors for biotic stresses, which significantly affect the pre-and post-harvest loss of crop yield. The insect and pest infestations reduced crop yield in rice maize, wheat, barley, oats, etc. during the pre-and post-harvest periods [77-82]. The post-harvest crop loss occurs from harvest to human consumption due to both biotic and abiotic factors. Scientists need to resolve this problem permanently through advanced crop breeding. It may help to develop a new tolerant variety to both biotic and abiotic factors and reduce yield loss.
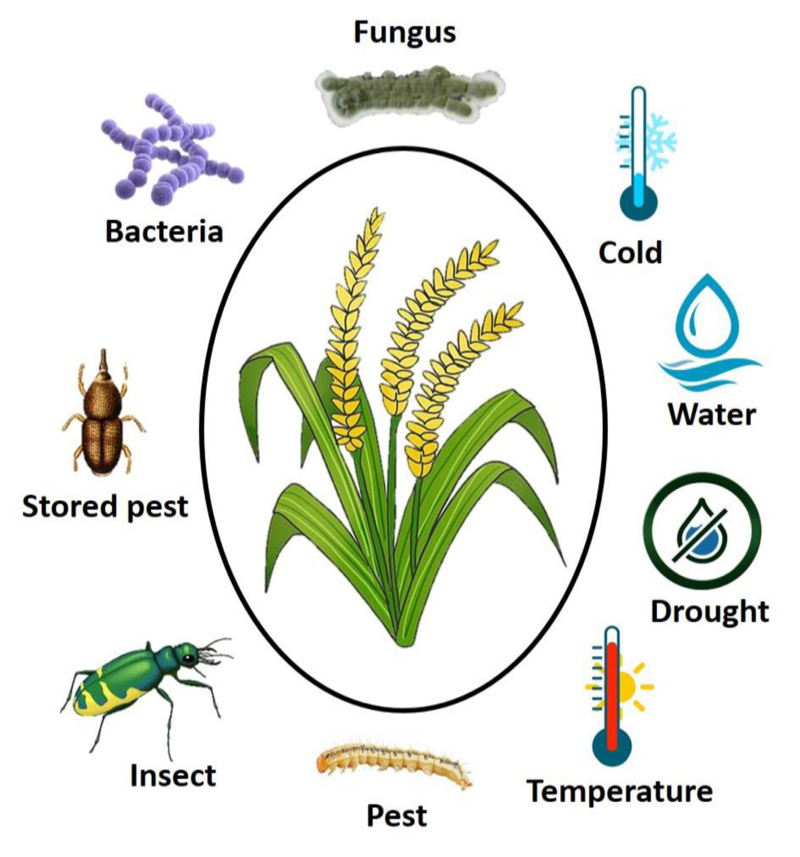
4. OVERVIEW OF CRISPR/CAS9 FOR CROP IMPROVEMENT
Gene editing uses engineered site-specific nucleases to remove, insert, or mutate a DNA sequence [83]. It is a recent tool used for crop breeding. Conventional breeding by genetic recombination or random mutagenesis is a time-consuming process. The advent of site-specific nucleases has highlighted the importance of site-directed mutagenesis over random mutagenesis [84]. It is very effective for improving the desirable traits of the crops. Advanced genome-editing techniques like zinc finger nuclease (ZFNs), transcriptional activator-like effect or nuclease (TALENs), and CRISPR/Cas9 offer platforms for transgene-free genome-editing and precisely target any gene of interest [85]. Among the genome editing techniques, CRISPR/Cas9 requires only a small piece of RNA (gRNA) to target any gene of interest [86]. It is a very efficient genome-editing system that improves crop traits under both biotic and abiotic stresses. The CRISPR/Cas9 system has been applied in many crop plants [87, 88]. The application of the CRISPR/Cas9 system in cereal crops was highlighted by Hillary and Ceasar [32]. The CRISPR/Cas9 genome-editing system paved the way for a nucleotide excision (remove, insert, or mutate) mechanism for crop improvement. It has high efficiency and accuracy which is highly helpful for crop improvement [89]. Genome-editing system like CRISPR/Cas9 has been considered to be a potential tool for crop improvement. In the last few years, developments in CRISPR/Cas9 system are spectacular and widely applied for target mutagenesis in crops, including gene knock-out and knock-in, modification, and suppression of target genes [88]. Despite the significant advances in CRISPR/Cas9 system, numerous limitations and concerns still exist, such as off-target mutations, indel mutations, and the absence of PAM sequence in the chosen gene loci [90]. These demerits bring concerns to the regulatory bodies, consumers, and the farmers to utilize CRISPR/Cas9 edited crops [91]. However, a variety of CRISPR-based systems (variants such as Cas12, Cas13, and Cas14, base editing, prime editing) are now in the row, which would serve as alternatives to Cas9 [92-96]. These advancements in CRISPR-based systems would definitely reduce the pre-and post-harvest yield loss in cereal crops and strengthen food security in the future.
5. APPLICATION OF CRISPR/CAS9 TO REDUCE PRE-AND POST-HARVEST YIELD LOSS
Cereals are the primary source of calories and nutrients and serve as a staple food for millions of people in developing countries. The pre-and post-harvest loss of crops is a serious issue for farmers to meet food production. The CRISPR/Cas9 system promises the rapid development of new varieties of crops with enhanced traits. The CRISPR-Cas9 genome-editing system has been successfully used to develop biotic and abiotic stress-resistant plants (Fig. 2).The various approaches for the reduction in pre-and post-harvesting loss of yield using the CRISPR/Cas9 system are discussed below.
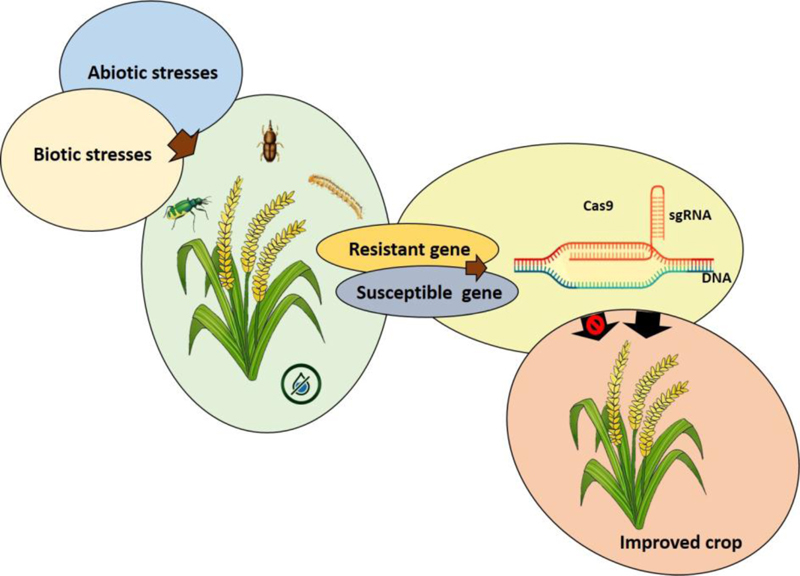
5.1. Reduction of Pre-Harvest Yield Loss by CRISPR/Cas9 System
Pre-harvest sprouting is a major problem in cereal crops. The moisture and temperature raise grain susceptibility to pre-harvest sprouting. The phytohormones like abscisic acid (ABA) and gibberellic acid (GA) are involved in seed germination and dormancy in many plants [97, 98]. These phytohormones have multi-functional properties. For example, the ABA is one of the multi-functional phytohormones, it is involved in many stress-induced responses. It has been shown that ABA plays a crucial role in regulating plant adaptation to various biotic and abiotic stresses, as ABA can trigger extensive changes in the transcriptome to help plants respond to environmental stresses [99-102]. The biosynthesis of these phytohormones is regulated by many genes. The knock-out or knock-in of target genes related to the biosynthesis of phytohormones will help reduce pre-harvest sprouting in cereal crops (Table 1). For example, OsABA2 gene codes for zeaxanthin epoxidase in rice which is involved in ABA biosynthesis [103]. Knock-out of the OsABA2 gene using the CRISPR/Cas9 system showed altered seed dormancy and germination in rice [104]. It may help to reduce yield losses in rice due to pre-harvest sprouting. The MOTHER OF FT AND TFL (MFT) gene is also involved in the regulation of ABA signaling-mediated seed germination. The OsMFT2 knock-out lines exhibited pre-harvest sprouting in rice, whereas pre-harvest sprouting did not exhibit in wild-type and OsMFT2 overexpression lines [105]. These results have given the insight into reducing the loss of pre-harvest sprouting in rice through the knock-in of OsMF2 genes through the CRISPR/Cas9 system in the future. The CRISPR/Cas9 system could be used to introduce desirable traits and reduce yield loss in crops. The qSH1 is an important gene associated with seed shattering in rice [106]. The knock-out of the qSH1 gene using the CRISPR/Cas9 system showed reduced grain shattering in rice [106]. Konishi et al. [107] reported that the loss of function of qSH1 gene in rice significantly improved strong seed shattering, which helps to improve the crops production. Many genes such as shattering abortion1 (SHAT1), shattering4 (SH4), growth-regulating factor 4 (GRF4), etc. have been identified in rice and they are responsible for rice grain shattering [108-110]. The CRISPR/Cas9 genome-editing system has allowed to improve the crop yield either by knock-in of resistant genes or knock-out of susceptible genes. The CRISPR-Cas9 system was used to mutate the salt and drought tolerance (DST) gene in rice [111]. Santosh et al. [111] revealed that the dst mutant lines improved salt and drought tolerance as well as grain yield in rice. Application of CRISPR/Cas system targeting these genes may help to improve the pre-harvest loss in cereals.
Table 1.
Name of the Crop | Type of Study | Target Gene | Functions/Description of Gene | Observation | Reference |
---|---|---|---|---|---|
Rice | Knock-out | qSH1 | Susceptible to seed shattering | Reduced the seed shattering | [106] |
Rice | Knock-out | MFT2 | Susceptible to sprouting | Reduced the post-harvest sprouting | [105] |
Rice | Knock-out | VP1and Sdr4 | Tolerance to sprouting | Increased the post-harvest sprouting | [98] |
Rice | Knock-out | ABA2 | Involved in ABA biosynthesis pathway | Enhanced the disease resistance and altered seed dormancy | [104] |
Rice | Mutation | Gn1a and DEP1 | Gene related to yield | Increased the grain yield | [132] |
Rice | Knock-out | PHS9 | Susceptible to sprouting | Increased the pre-harvest sprouting | [133] |
Rice | Mutation | SD1 | Tolerance to lodging | Increased the resistance to lodging | [134] |
Rice | Mutation | DST | Tolerance to abiotic stress | Increased the tolerance to salt and drought stress | [111] |
Rice | Mutation | SAPK2 | Tolerance to drought | Increased the tolerance to drought stress | [135] |
Rice | Knock-out | Ann3 | Tolerance to cold | Increased the tolerance to cold stress | [136] |
Rice | Knock-out | SWEET13 | Susceptible to blight disease and involved in sugar synthesis | Increased the resistance to bacterial blight disease | [113] |
Rice | Mutation | ERF922 | Tolerance to pathogenicity | Increased the resistance to pathogenicity | [118] |
Rice | Mutation | SEC3A | Tolerance to blight disease | Increased the resistance to blight disease | [137] |
Rice | Knock-down | CYP71A1 | Involved in biosynthesis of serotonin | Increased the resistance to brown plant hopper | [122] |
Wheat | Knock-out | MLO | Susceptible to powdery mildew | Increased the resistance to powdery mildew |
[114] |
Wheat | Knock-out | EDR1 | Susceptible to powdery mildew | Increased the resistance to powdery mildew |
[117] |
The CRISPR/Cas9 system was also successfully applied in biotic stress management in cereal crops (Table 1). Pre-harvest yield losses due to diseases can be up to 15% in major food crops [31]. Bacterial blight is a major disease (caused by Xanthomonas oryzae pv. Oryzae) that constrains rice production in Asia [112]. The knock-out of the OsSWEET13 gene using the CRISPR/Cas9 system showed improved resistance to bacterial blight disease in rice [113]. The knock-out of OsSWEET13 reduces the sugar availability within the xylem vessels and prevents bacterial colonization in rice. This may help to reduce the crop yield losses due to bacterial blight disease. The fungus is responsible for multiple diseases including mildew, rot, rust, and smut, which can cause huge yield loss in crops. The mildew-resistance locus (MLO) is one of the susceptible genes to powdery mildew in wheat. Knock-out of mildew-resistance locus (TaMLO) gene using the CRISPR/Cas9 system showed resistance to powdery mildew disease in wheat [114]. As per a very recent breakthrough study, TaMLO-R32 mutant lines created by a multiplex CRISPR system maintain the normal growth and yields as well as confer resistance to powdery mildew disease in wheat [115]. Editing of MLO gene in wheat may provide the opportunity to breed varieties with improved grain yield [114, 116]. CRISPR/Cas9 system-mediated knock-out of enhanced disease resistance1 (EDR1) resulted in enhanced resistance in wheat to powdery mildew caused by Blumeria graminis [117]. In rice, the knock-out of the ethylene-responsive factors 922 (ERF922) gene by the CRISPR/Cas9 system showed improved resistance to pathogenicity [118]. The CRISPR/Cas9 system is very useful for the functional characterization of target genes. So far, many biotic and abiotic stress-responsive genes have been identified in crops. Most of the genes are very helpful for reducing the pre-harvest loss of grain yield. The knock-out or knock-in of stress-responsive genes through the CRISPR/Cas9 system could help develop improved crop variety. It may improve the economic status of farmers and reduce the food demand in the coming decades.
5.2. Reduction of Post-Harvest Yield Loss by CRISPR/Cas9 System
Insects and pests mainly affect post-harvest yield loss in cereals. Improving the quality of the grains could help develop resistance against the damage caused by insects and pests. The CRISPR/Cas9 genome-editing system has allowed the development of new insect-pest resistant crop varieties by either knock-in insect-pest resistant genes or knock-out of susceptible genes (Fig. 2). The chemical and physical properties of grains are responsible for defense mechanisms against insect-pest damage [119, 120]. The chemical composition of grain cell walls, the color of the testa,, glume and grain phenol content, etc. contribute to grain insect-pest attack as well as mold resistance [121]. Alteration of grain components could be effective for reducing the post-harvest loss of grain yield. These strategies maybe effectively utilized in the CRISPR/Cas9 genome editing for insect-pest control. For example, the inactivated CYP71A1 gene prevents serotonin biosynthesis and increases the salicylic acid content in plants, which helps to improve insect-resistant and prevent yield losses [122]. CYP71A1 knock-out mutants prevented serotonin synthesis and conferred disease-resistance in rice [123]. CRISPR/Cas9-mediated knock-down of CYP71A1 gene in rice showed resistance to brown planthopper [122]. So far, many insect-pest resistant genes have been identified in crop plants [124, 125]. Genome-editing in insect-pest with CRISPR/Cas9 system has been recognized as a potential tool for insect-pest control [126]. The CRISPR/Cas9 system-mediated knock-out of various genes of insect-pest such as Spodoptera litura [127, 128], Spodoptera littoralis [129], Helicoverpa armigera [130], and Plutella xylostella [131] helped to protect the plants from insect damage. CRISPR/Cas9 system has great prospects in controlling agricultural insects and pests (Table 1).
CONCLUSION AND FUTURE PROSPECTS
The economy of the developing country is mainly dependent on agriculture and the large-scale production of crops. Pre-and post-harvest loss is a major issue in the agriculture sector. Both biotic and abiotic factors influence crop production. The agricultural management and adequate storage facilities cannot reduce the pre-and post-harvest yield loss. It is not effective in the long term due to current societal and climatic changes. Developing crop varieties with increased shelf life is an important approach in reducing the pre- and post-harvest loss of yield. Many advanced approaches are available for crop improvement. Genome-editing is one of the most important solutions for crop improvement. It helps to alter the desirable traits of the crops. It is a long-term solution for reducing pre-and post-harvest yield loss. In the current scenario, transgenic-free crops are safe for human health. The CRISPR/Cas9 system offers a precise transgene-free genome-editing approach. This approach offers new opportunities for addressing pre-and post-harvest loss of crop yield. It is widely used for improving crop traits. The CRISPR/Cas9 system is an asset for crop improvement. It can help to reduce yield loss and strengthen food security in the future.
AUTHORS' CONTRIBUTIONS
TPAK, TM, and SAC conceptualized and wrote the manuscript. SAC critically revised the manuscript for publication.
CONSENT FOR PUBLICATION
Not applicable.
FUNDING
This work was financially supported by Rajagiri College of Social Sciences (Autonomous), Kerala, India, under Seed Money for Faculty Minor Research.
CONFLICT OF INTEREST
The author declares no conflict of interest, financial or otherwise.
ACKNOWLEDGEMENTS
We sincerely thank Rajagiri College of Social Sciences, Kochi, Kerala, for providing the research facilities and support.