All published articles of this journal are available on ScienceDirect.
Phyto-fabrication of Cobalt Oxide Nanoparticles from Ocimum Gratissimum L. Leaf and Flower Extracts and their Antimicrobial Activities
Abstract
Background:
Phyto-fabrication of nanoparticles has gained attention in recent times owing to its simple mode, cost-effective and eco-friendly nature.
Objective:
Hence, the present study aimed to synthesize cobalt oxide nanoparticles from methanol extracts of Ocimum gratissimum flower and leaf and to evaluate their antimicrobial action towards pathogenic bacteria and fungi.
Methods:
Phyto-fabrication of Cobalt nanoparticles (CoONPs) was achieved using cobalt chloride hydrate solution as a precursor. Characterization of fabricated CoONPs was performed using Ultra Violet-Visible spectrometry (UV-Vis), X-ray diffractometer (XRD), and Fourier Transform-Infrared spectroscopy (FTIR). The antimicrobial property was tested against two bacteria (Staphylococcus aureus and Escherichia coli) and two fungi (Cryptococcus albidus and Candida globasa) by agar disc diffusion technique and by measurement of Minimum Inhibitory Concentration (MIC).
Results:
Initial confirmation of CoONPs synthesis was observed by the colour change from light pink to reddish pink. Further, the UV-Vis spectrophotometry validated the CoONPs synthesis with a peak at 509 nm. The XRD authenticated the crystal nature of CoONPs synthesized using flower extract at 2θ angles with an average size of 54.9 nm and CoONPs fabricated using leaf extract at 2θ angles with an average size of 55.02 nm. The FTIR spectroscopy confirmed the functional groups present in plant extracts, which are believed to reduce and stabilize CoONPs. The findings of antibacterial activity showed that CoONPs synthesized using leaf showed a higher inhibition zone against E. coli (20.00 ± 2.00 mm) than S. aureus. Relating to fungi, the CoONPs fabricated using flower extract displayed significantly the highest activity against C. albidus (28.67±0.57 mm) and C. globasa (25.0 ± 0.00 mm). The lowest MIC was observed against S. aureus (MIC 7.5 µg/ml). For fungi, the smallest MIC was found against C. albidus (MIC 2.5 μg/ml).
Conclusion:
The current research established the efficacy of the phytochemical constituents of leaf and flower extracts of O. gratissimum for the enhancement of the effectiveness of CoONPs against both the tested bacteria and fungi.
1. INTRODUCTION
The bacterial and fungal resistance to conventional antibiotics and medicines has persistently emerged. Hence, there's a dire need to develop alternative antimicrobial agents that could end the menace of bacteria and fungi that are resilient to existing antibiotics [1]. To overcome this, there are promising alternatives to combat pathogenic microbes through nanotechnology by synthesizing nanoparticles [2]. Amidst various metal nanoparticles, cobalt oxide NPs are known to sustain astonishing physical, chemical, and optical properties, at extreme circumstances [3]. Cobalt, a constituent of vitamin B12, is useful for the treatment of anemia since it initiates the red blood cells formation [4]. Moreover, it is used in the arena of Nanosensors and Nanoelectronics owing to its unique optical, magnetic, catalytic, and electrical properties [3, 5]. Various states of oxidation or multivariate states such as Co2+, Co3+, and Co4+ can be exhibited by cobalt, which is responsible for its diverse states of spin in their oxide forms, i.e., low, intermediate, and high. This feature allows cobalt to be used for different industrial purposes [6].
The biomedical industry has embraced the applications of CoONPs that are used as antimicrobials, for heartburn problems, regeneration of bone, drug delivery, as well as for cancer treatment [7]. Many approaches, such as the physical, chemical, and biological techniques, are reported for the fabrication of CoONPs [8]. Among the prevailing methods, chemical methods are capable of synthesizing great amounts of nanoparticles with controlled distribution and size in a comparatively short time [1]. However, the usage of chemicals with high toxicity, the production of harmful wastes, and high energy consumption are the demerits. Similarly, physical methods such as the microwave technique and cluster beam deposition, with the contribution of high temperature, high pressure, and radiation, are also used for the fabrication of nanomaterials [9]. Due to this, methods utilizing biological materials are the better replacement for the above-said methods for the fabrication of nanoparticles [10].
Nowadays, various microorganisms and plant extracts are used effectively for the fabrication of metal NPs either intracellularly or extracellularly [10, 11]. The bio-method is a bottom-up approach and requires less energy, less wastage of ingredients and a proper control of reagents. Hence, nowadays efforts are made to evaluate the antibacterial effects of bio-synthesized nanoparticles and the synergistic effect of both the metal and the plant extracts [12]. Above all, phyto-synthesis of nanoparticles is highly preferred as this method is cost-effective, efficient, eco-friendly, and involves a comparatively smaller amount of energy than physical and chemical approaches [13]. The nanomaterials fabricated by biosynthesis are well known for theirsmaller size, dynamic movement, and reduced surface area [14]. Moreover, they exhibit fewer side effects than the synthetic antibiotics [13]. Bio-fabrication of CoONPs has been given utmost interest due to economical production and various electrical, mechanical and optical properties of the synthesized cobalt NPs [12]. Above all, CoONPs are less toxic at low concentrations in the human body and have potent activities towards pathogenic microbes at lower doses. Recent research established the biosynthesis of CoONPs and their efficacy against microbial pathogens [15-18]. The sweet basil (O. gratissimum L.) is a medicinal plant endowed with active components that are useful against various pathogenic conditions. Moreover, studies on the synthesis and antimicrobial activities of nanoparticles in Ethiopia are in the juvenile stage. Therefore, this study was carried out to investigate the phyto-fabrication of CoONPs from leaves and flowers of O. gratissimum and assess their antimicrobial activities.
2. MATERIALS AND METHODS
2.1. O. Gratissimum Leaf and Flower Extracts Preparation
The fresh leaves and flowers of O. gratissimum were collected from the locality in Damota village, Haramaya district, Ethiopia. The fresh O. gratissimum leaves and flower materials were washed 3 times with water to remove dust and were dried before being powdered. After that, the dried leaves and flower materials were powdered using a homogenizer. Then 20 g of powder samples were added to 200 ml of methanol in two flasks, separately. Then, they were kept in a water bath shaker for 8. Thereafter, the crude extracts with solvent were filtered with the help of Whatman No.1 filter paper to remove particulate matter and to obtain the methanol extract of O. gratissimum. Freshly prepared methanol extract of O. gratissimum leaves and flowers were sealed with aluminum foil. Then, it was stored at 4 °C for future use.
2.2. Phyto-fabrication of CoONPs
The CoONPs fabrication was performed using Cobalt chloride hexahydrate (CoCl2 6H2O) as a precursor [19]. Aqueous solution 1.06 x 10-2 M of CoCl2 6H2O was prepared in 250 ml Erlenmeyer flask, and 20 mL of plant parts extracts of O. gratissimum (leaves, flowers) were separately added drop wisely to 250 mL conical flask containing l00 ml of 1.06 x 10 -2 M CoCl2 6H2O solution for the reduction of Co+ ions. The complete mixture was kept on magnetic stirring at 700C temperature. Suitable controls were maintained throughout the conditions of the experiments. The mixture was then centrifuged at 4500 rpm for 15 min. The precipitates obtained were kept in an oven (500 °C) for 2h, grounded, and subjected to characterization by different spectrophotometric analyses.
2.3. Characterization of CoONPs
2.3.1. Visual and UV–Vis spectroscopy examination
The phyto-synthesis of CoONPs using O. gratissimum (leaves, flowers) in the medium was initially confirmed by the changes in color from a light pink to reddish brown . Besides, characterization of the formation of biosynthesis of CoONPs and bio-reduction of the Co+ ions in solution was monitored by UV-visible spectroscopy (Model 6705) at the wavelength of 200-700 nm range. The absorption wavelength at maximum was measured by keeping each aliquot NP material in a quartz cuvette (1 x 1 cm) functioning at a resolution of 1 nm, using distilled water as the blank. The wavelengths of the Co nanoparticles were observed based on the SPR formed for the metal, which is produced by clear oscillation of electrons generated by a light source [20].
2.3.2. Characterization by XRD
Characterization by XRD was done using X-ray diffractometer (XRD-7000, SHIMADZU, Japan) with monochromatic radiation (Cu kα; λ = 1.5406 Å) functioned at 30 mA and 40 kV at a pattern of 2θ angle. The range of 20º–80º was used for scanning and the images acquired were equated with the Joint Committee on Powder Diffraction Standards (JCPDS) library to determine the crystal structure [20]. The size of the NP grain was calculated with the help of Scherer’s Formula as follows:
D = (0.9Xλ) /βXCosθ
Where, D denotes Average Crystal size, β denotes Line broadening (radians), θ denotes Bragg angle and λ denotes wavelength of X-ray.
2.3.3. FTIR Characterization
The FTIR analysis was performed by Fourier Transform Infrared Spectrophotometer (model: Perkin Elmer Frontier FTIR) [21]. The analysis was done to detect the chemical groups that might be responsible for the capping and reduction of the Cobalt bulk materials to NPs at a wavelength of 4000- 400 cm-1 range. For this, the dried sample was added with Potassium Bromide (KBr) and it was made to 1 mm thickness which was appropriate for FTIR analysis.
2.4. Antimicrobial Activity of Phyto-fabricated CoONPs
The antimicrobial effect of Cobalt NPs was investigated using disc diffusion and MIC methods [22]. In the disc diffusion method, three concentrations (15, 25 and 35 μg/ml) were used against two different bacterial species (gram-positive Staphylococcus aureus and gram-negative Escherichia coli) and two fungi (Cryptococcus albidus and Candida globasa). The two test bacteria were procured from EPHI, the Ethiopian capital city of Addis Ababa. The two fungal pathogens were obtained from the Plant Pathology lab at Haramaya University. The bacterial and fungal stains were sub-cultured and maintained on Nutrient Agar and Potato Dextrose Agar (PDA), respectively. The bacterial culture was adjusted to the turbidity of 0.5 McFarland standard solutions (1.5×108 bacteria/ml). Henceforth, the bacterial and fungal cultures were incubated for 18-24 h at 37 ºC and 72 h at 27 ºC respectively. For the determination of minimum inhibitory concentration (MIC), broth dilution method was employed.
2.4.1. Agar Disc Diffusion Test
For antimicrobial activity of O. gratissimum synthesized CoONPs by disc diffusion method, bacterial and fungal cultures (200 µl) were uniformly streaked as lawns on the respective medium. Ten µl of the CoONPs at three different concentrations (15, 25 and 35 μg/ml) were loaded into Whatman No. 1 paper discs (6 mm), and the discs were accommodated on the lawns of bacterial and fungal cultures. Amoxicillin (10 µg/ml) and ketoconazole (10 µg/ml) were utilized as positive controls for bacteria and fungi, respectively whereas, methanol was used as a negative control. The Petri plates were then incubated for 24 hr at 37°C for bacterial strains and 28°C for 72 hr for fungal strains. After incubation, the zones of inhibition of the pathogens were measured.
2.4.2. MIC Determination
For MIC determination, the lowest concentrations of CoONPs that displayed good antimicrobial activity during the agar diffusion method were selected for the serial dilution method and the method of Adera et al. [23] was followed with slight modifications. In brief, two-fold dilution of 15 µg/ml, 7.5 µg/ml, 3.75 µg/ml, 1.875 µg/ml, and 0.9375 µg/ml from 15 µg/ml of CoONPs. Nutrient broth and potato dextrose broth (2 ml) were added for bacteria and fungi respectively into test tubes and CoONPs (0.1 ml) were mixed with the nutrient broth and potato dextrose broth. Afterwards, Inoculums (0.1 ml) of each pathogen were distributed into the test tubes comprised of broth suspensions and the CoONPs. The test tubes were then closed using aluminum foil and incubated at 37°C for 24 hrs in the case of bacteria and 27°C for 72 hrs for fungi. Finally, the tubes were monitored for presence or absence of the visible growth of the microbes. The least concentration at which no visible of the microbes noted was the value of MIC. The MIC test was done in triplicate for each pathogen.
2.5. Data Analysis
The experimental data in Tables 1 and 2 (supplementary material file) was first entered into Microsoft Excel, and a one–way analysis of variance (ANOVA) was used from 9.2 software package (SAS version), and the statistical results were reported as a mean ± standard deviation. Using Post Hoc' DMRT, significant differences were specified at P<0.05.
Plant Extract | 2θ (deg.) | FWHM | D (nm) |
---|---|---|---|
Flower | 40.90221 | 0.1567 | 54.90913 |
Leaf | 39.77866 | 0.156738 | 55.02112 |
Pathogens | Part of the Plant Used | The Concentration of Biosynthesized Nanoparticles (µg/ml) | Amoxicillin (10 µg/ml) | Met | ||
---|---|---|---|---|---|---|
15 µg/ml | 25 µg/ml | 35 µg/ml | ||||
E. coli | Leaf | 11.33 ± 1.15cdD | 14.33 ± 0.57Dc | 20.00 ± 2.00cB | 19.67 ± 0.57aA | - |
E. coli | Flower | 11.67 ± 0 .57cdC | 13.67 ± 0.57dC | 19.67 ± 2.51bC | 20.5 ± 1.30aA | - |
S. aureus | Leaf | 10.33 ± .0.57dD | 12.33 ± 0.57dC | 16.00 ± 0.00dB | 18.83 ± 1.04aA | - |
S. aureus | Flower | 10.00 ± 0.00Dd | 12.33 ± 0.57dC | 15.00 ± 1.00dB | 19.83 ±1.32aA | - |
- | - | - | - | Ket (10µg/ml) | Met | |
C. albidus | Leaf | 21.00 ± 1.00aD | 23.67 ± 0.57aC | 28.33 ± 1.52aB | 30.5 ± 0.57aA | - |
C. albidus | Flower | 18.67 ± 2.30aC | 25.33 ± 0.57aC | 28.67 ± 0.57aA | 31.17 ±1.04aA | - |
C. globasa | Leaf | 13.67 ± 1.50bcC | 17.67 ± 0.57cB | 23.67 ± 2.05bA | 29.83 ± 0.16aA | - |
C. globasa | Flower | 14.67 ± 0.5bC | 21.3 ± 2.3bB | 25.0 ± 0.00abA | 28.0 ± 1.00aA | - |
3. RESULTS
3.1. Characterization of Synthesized CoONPs
3.1.1. Visual Observation of Synthesized CoONPs
The biosynthesis of CoONPs from methanol extract of leaves and flowers of O. gratissimum was, to begin with, affirmed by visual perception of the change of the color amid response (Fig. 1). The aqueous solution of CoCl2 6H2O was shown light pink, which was later changed to reddish brown as we added extract. This color change demonstrated the formation of CoONPs, whereas controls (without Co+ particles) showed no alteration in color.
3.1.2. Characterization based on UV-visible Spectro-photometry
The brown-colored samples of CoONPs synthesized using both the leaves and flower extracts of O. gratissimum were subjected to UV-Spectrophotometry in the range of 200-700 nm. Fig. (2A and B) indicated the absorbance crest obtained for phyto-fabricated CoONPs.
3.1.3. Characterization based on (XRD)
The XRD analysis of the phyto-fabricated Cobalt oxide NPs from the leaves and flower extracts of O. gratisimmum was recorded and a typical XRD pattern is shown in Fig. (3A and B). The high diffracted intensities were obtained around 2θ = 16.08°, 20.7°, 31.3°, 33.04°, 37.82°, 38.88°, 39.32°, 42.28°, 43.58°, 50.56°, 54.64°, 55.24°, 67.98°corresponding to the plane 110, 020, 111, 220, 120, 130, 201, 422, 040, 131, 400, 420, 440 for A and 16.06°, 20.78°, 31.32°, 32.46°, 33.00°, 33.82°, 37.8°, 38.84°, 39.28°, 42.26°, 43.56°, 55.24°, and 58.04° corresponding to the plane 110, 020, 111, 220, 120, 130, 201, 422, 040, 131, 400, 420, 440 for B. Bragg reflections of Face centered (FCC) crystalline phases of the Cobalt NPs and they are in agreement with the pattern no. 96-152-4727.
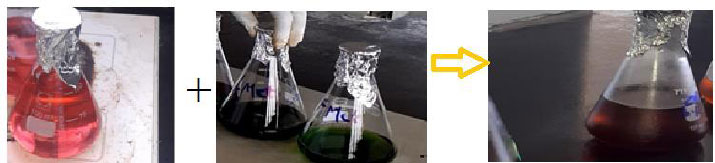
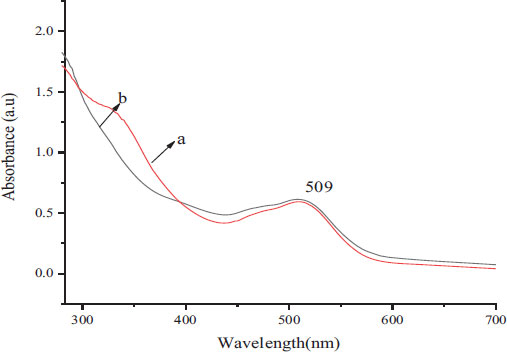
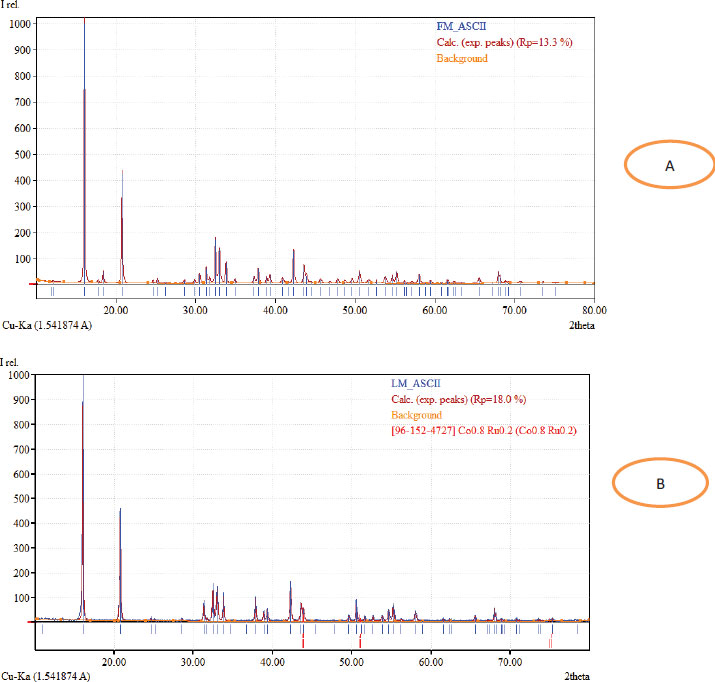
The XRD peaks measured were found to match with the Cobalt’s standard patterns. The sharp peaks authenticated the crystal nature of CoONPs. The average crystal size of fabricated NPs with the flower extract was measured to be 55.02 nm based on the equation of Debey-Scherrer. The average size of 54.90 nm was observed for CoONPs synthesized with the leaf extract as it is shown in Table 1.
3.1.4. Characterization by Fourier Transformation Infrared (FTIR) Spectroscopy
Fig. (4a and b) showed the FTIR result of CoONPs recorded inside the wave number run 500-4500 cm-1. The FTIR spectra of CoONPs from leaves and flowers extract of O. gratissimum observed different bands at 667, 1050, 1386, 1425, 1625, 3439 and 478, 669.79, 778, 1051, 1341.9, 1419.9, 2170, 3439 cm-1 respectively. These compared to Co–O bowing mode of CoO in Co was Co2+ and was tetrahedrally coordinated.
FTIR spectra of leaves-mediated biosynthesized cobalt oxide nanoparticles showed the two-solid band 3439 cm-1 and 1,341.9 cm-1. The broadband at 3439 cm-1was due to O-H extending in alcohols and phenols or N-H extending of amines. The top at 1,341.9 cm-1 was compared to the essential and auxiliary amine bunches of N–H bowing vibrations of proteins and characteristic of –C=O carbonyl bunches. The crests at 2,170 cm-1 related to alkynyl C≡C stretch and crests at 1,051 cm-1 may well be attributed to –C–O, –C–O–C extending, and C-N stretch of aliphatic and aromatic amine. The pick at 669.5 cm-1indicated the nearness of Co–O vibrations in which Co is Co 2+ and is tetrahedrally coordinated.
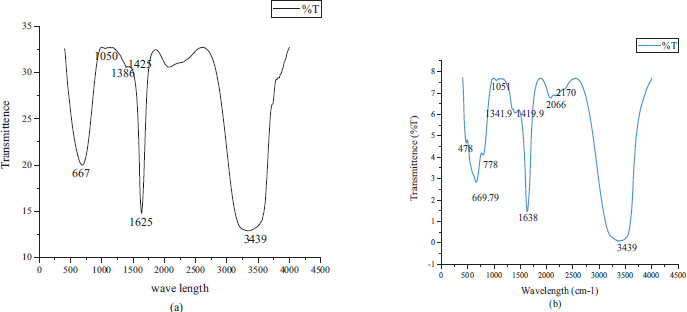
3.2. Antimicrobial Activity of CoONPs
3.2.1. Antibacterial Studies
Antibacterial activity of biosynthesized CoONPs from O. gratissimum leaf and flower extracts at three different concentrations is recorded in Table 2. The findings revealed that the antibacterial action of CoONPs was concentration-dependent. The results exhibited higher activity by leaf-fabricated CoONPs against E.coli followed by S. aureus with a zone of inhibition of 20.00 ± 2.00 mm and 16.00 ± 0.00 mm, respectively. The flower extract-fabricated CoONPs displayed significantly (P<0.05) higher activity against E.coli (19.67 ± 2.51 mm) than S. aureus (15.00 ± 1.00 mm). The antibacterial potential of CoONPs was found to be significantly (P<0.05) less than that of the standard antibiotic amoxicillin. Methanol, as a negative control, did not show any antibacterial activity.
3.2.2. Antifungal Activity of Biosynthesized CoONPs
The results of in vitro antifungal activity of CoONPs fabricated using leaves and flowers of O. gratissimum against C. albidus and C. globasa are illustrated in Table 3 and Fig. (5). The CoONPs exhibited significant antifungal activity against the tested fungi (C. albidus and C. globasa). The leaf-fabricated CoONPs established the highest antagonism towards C. albidus and C. globasa with the inhibition diameter of 28.33±1.52 and 23.67 ± 2.05 mm respectively at the concentration of 35 μg/ml. The flower-fabricated CoONPs demonstrated the highest activity against C. albidus and C. globasa with a zone of inhibition of 28.67±0.57 mm and 25.0 ± 0.00 mm respectively at the concentration of 35 μg/ml. The antifungal potential of ketoconazole against the tested fungi was found to be significantly higher than the activity (P<0.05) of CoONPs with an inhibition zone range of 31.17±1.04 mm to 25.00±1.00 mm C. albidus and C. globasa against respectively.
Pathogens | Part of the Plant | Amoxicillin/Ketoconazole (µg/ml) | MIC of CoONPs (µg/ml) |
---|---|---|---|
S. aureus | Leaves | 5.00 ± 0.00 | 12.50±4.33 |
Flowers | 15.00±0.00 | ||
E. coli | Leaves | 5.00 ± 0.00 | 7.50±0.00 |
Flowers | 10.00±4.33 | ||
C. albidus | Leaves | 1.75±0.00 | 2.50±1.08 |
Flowers | 2.50±1.08 | ||
C. globasa | Leaves | 2.5±0.00 | 3.75±0.00 |
Flowers | 3.75±0.00 |
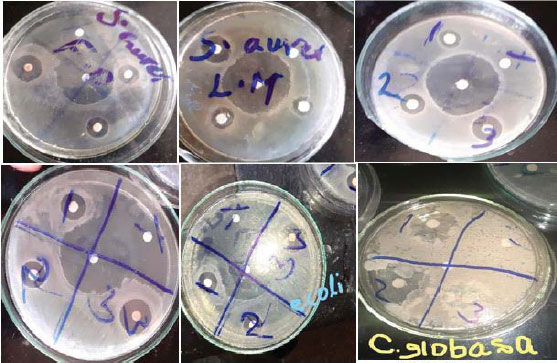
3.2.3. The Minimum Inhibitory Concentrations of CoONPs
Furthermore, the antimicrobial potential was evaluated to determine the MIC of phyto-fabricated CoONPs against the tested pathogenic microorganisms using the serial dilution method. The MIC values of the biosynthesized CoONPs from O. gratissimum leaf and flower methanol extract are recorded in Table 3.
In the current investigation, the lowest MIC (7.5 µg/ml) and the highest antibacterial potential of the CoONPs was noted against S. aureus, while a lesser potent antibacterial activity with MIC value (15 µg/ml) was observed towards E. coli. Besides, the highest antifungal effect by CoONPs was found against C. albidus (MIC 2.5 μg/ml) and the lowest antifungal activity against C. globasa with a MIC value of 3.75 μg/ml.
4. DISCUSSION
As the research on nanoparticles is fresh-faced stage in Ethiopia, the current work was carried out on phyto-assisted fabrication of CoONPs using the flower and leaf extracts from O. gratissimum. Post-synthesis, the CoONPs were subjected for validation using characterization techniques and antimicrobial activity against two bacterial and two fungal pathogens.
The initial validation of CoONPs was observed by light pink to reddish brown color change in the reaction mixture which suggested that the precursor was reduced to Cobalt ions (Co+) with the help of phyto-components of the flower and leaf extracts. The reddish brown color formation was attributed to the occurrence of External Plasmon Resonance [24]. Further, the UV spectroscopic examination for the fabricated CoONPs disclosed a 590 nm peak that demonstrated a nearness of nanoparticles within the response blend. The CoONPs would generally display a band of absorption at 350-550 nm range which is accredited to Surface Plasmon Resonance of Co nanostructure [25]. The present results endorse the preceding absorption spectral profile [25]. The alteration in the color of the response blend was moreover due to the surface Plasmon resonance that provides a helpful sign of the arrangement of CoONPs.
With regard to the characterization of CoONPs by XRD, the planes detected suggested that the phyto-fabricated CoONPs were crystal in nature. The scattering peaks at 2θ angle were indexed at different planes as shown in the results. The results obtained in our study were in concomitant with the results reported by Khalil et al. [26]. Additionally, certain unassigned peaks found at the angle of 2θ might be accredited to impurities in the sample or attributed to crystals of organic contents [27]. Albeit the paramount role of electron microscopy in the determination of the size and shape of the CoONPs, the lack of facilities hampered us to perform the same.
The FTIR examination was utilized to decide the biomolecules that are responsible for capping and proficient stabilization of cobalt oxide nanoparticles synthesized utilizing O. gratissimum leaves, and flowers extract. FTIR can identify functional groups present inside the nanoparticles synthesized using plant and its parts extracts. The FT-IR spectra obtained were recorded for CoONPs between 4500 - 500 cm-1 in KBr pellets at the resolution of 4 cm−1. The wide band at 3439 cm-1was the characteristic crest of a hydroxyl (O-H) bunch of phenolic compounds [28]. The absorption band observed at 1386 cm-1 was owing to the C-O extend whereas the absorption band at 1625 cm-1 was because of C=C and C=O extension of benzene and amide-I linkage. The exceptionally solid band at 1050 cm-1emerged from C-O-C symmetric extending, C-O in carbohydrates, ester, liquor, ether, and C-O-H twisting vibrations of protein in O. gratissimum. Retention crests within the FTIR range of CoONPs showed up at 669 cm-1, compared to the metal-oxygen extending vibration. The observed bands may be considered for the proximity of various compounds such as phenolic, flavonoid, and carboxylic compounds. All of these asserted that methanol-soluble phytochemicals displayed within the leaves and flower extract of O. gratissimum can achieve reduction and stabilization of the cobalt nanoparticles [29]. Huang et al. [30] reported, on MNP blend, the useful bunches of alkaloids, anthracenes, flavones, and, such as –C–O–C–, ––C═C–, C–O–, and –C═O–.
The present study highlighted the potential of CoONPs against E. coli and S. aureus. S. aureus is considered to be the reason for the infection of soft tissues, bacteremia, and pneumonia, and has been stated to be resistant to methicillin [31]. The inhibitory potential of the CoONPs against the bacteria tested was owing to the large surface area to volume ratio of the nano-sized particles that enhanced their interaction with the microorganisms and caused a change in their permeability. This permeability allowed the NPs to enter into bacterial cells and directly kill them [32]. The CoONPs could directly bind to the bacterial DNA to induce cell death of the bacteria. The reduced CoONPs were capable of binding directly with the bacterial DNA to encourage cell death of the bacteria [33]. Similar studies were found to exhibit the effect of CoONPs against gram (-) E.coli and gram (+) S. aureus [17, 34-36]. The CoONPs at all three concentrations exhibited higher inhibition zones against E. coli than against S. aureus. A similar trend was reported in previous research by [37]. The reason for the distinctive sensitivities of gram (-) and gram (+) bacterial pathogens to the biosynthesized CoONPs could be due to the morphological contrasts between these microorganisms [38]. Besides, the positive ions of Cobalt NPs {Co (2+)} interact directly with the negatively charged liposaccharides found on the cell surface of Gram-negative bacteria that lead to the inhibition of enzyme biosynthesis [39]. The current study also revealed that CoONPs displayed antifungal activity against the fungi C. albidus and C. globasa. The effect of CoONPs against the fungi showed that the Cobalt NPs were able to stabilize therapeutic components from plant extracts that could penetrate extracellular polymeric substances (EPS) to kill fungal cells [40].
CONCLUSION
The phyto-fabrication of CoONPs using plant extracts assured a quick, less-toxic, and eco-friendly path. The current study showed the action of methanol extracts in O. gratissimum leaves and flowers for the fabrication, capping, and stability of CoONPs. The phyto-fabricated CoONPs were characterized effectively with UV-Vis spectra, FT-IR and XRD methods. Effective antibacterial action of the phyto-fabricated CoONPs towards E. coli and S. aureus and antifungal activity against C. globasa and C. albidus were detected. Based on the observed findings, the current research would be an attempt to further carry out investigations in the future on the efficacy of CoONPs synthesized using Ethiopian medicinal plants against broad spectrum of microbial pathogens that exhibit resistance to conventional antimicrobial drugs. Future studies are also recommended to explore medicinal plants from Ethiopia for phyto-synthesis of other nanoparticles to combat microbial infections.
AUTHORS’ CONTRIBUTION
Mohammed Abdi contributed to Initiation and design of the study, Lab experiment, and data analysis; Zekeria Yusuf contributed to lab experiment, data collection, and write-up of the document; J.M. Sasikumar: wrote the manuscript, and contributed to analysis and interpretation of data. All authors contributed to drafting the article and revising it critically for important intellectual content.
LIST OF ABBREVIATIONS
CoONPs | = Cobalt nanoparticles |
UV-Vis | = Violet-Visible spectrometry |
XRD | = X-ray diffractometer |
MIC | = Minimum Inhibitory Concentration |
JCPDS | = Joint Committee on Powder Diffraction Standards |
KBr | = Potassium Bromide |
PDA | = Potato Dextrose Agar |
ETHICS APPROVAL AND CONSENT TO PARTICIPATE
Not applicable.
HUMAN AND ANIMAL RIGHTS
No humans/animals were used for studies that are the basis of this research.
CONSENT FOR PUBLICATION
Not applicable.
AVAILABILITY OF DATA AND MATERIALS
The CoO nanoparticles manuscript data file used to support the findings of this study is included within the supplementary information file(s).
FUNDING
This project was funded by the Haramaya University Research grant, under project code: HURG_2020_06_01_98
CONFLICT OF INTEREST
The authors declare no conflict of interest.
ACKNOWLEDGEMENTS
The authors are grateful to the Haramaya University Research Office for their financial support and Laboratory facility.
SUPPLEMENTARY MATERIALS
The CoO nanoparticles manuscript data files used to support the findings of this study are included within the supplementary information file(s).