All published articles of this journal are available on ScienceDirect.
A Cisgenic Approach in the Transformation of Bread Wheat cv. Saratovskaya 29 with Class I Chitinase Gene
Abstract
Background:
Bread wheat is one of the major crops grown worldwide, showing high demand for new varieties with traits such as pathogen resistance. As the public acceptance of transgenic plants remains low, a novel approach – cisgenesis – is being developed to introduce the genes from the same or closely related species.
Objective:
This study presents a cisgenic approach used for the transformation of wheat with class I chitinase gene derived from T. aestivum cv. Stepnaya 15, co-transformed with acetohydroxyacid synthase gene that provides tolerance to imidazolinone herbicides.
Methods:
Calli from immature embryos of spring bread wheat Triticum aestivum cv. Saratovskaya 29 were used for co-transformation with two independent Minimal Expression Units (MEUs): class I chitinase and Acetohydroxyacid Synthase (AHAS) gene. For identification of cisgenic plants, genomic DNA was extracted from the leaves of imazethapyr-resistant regenerant plants at the plantlets stage and screened by polymerase chain reaction. The efficiency of transformation was calculated as the relation of regenerated plants with chitinase gene insert to the total number of calli in the experiment.
Results:
The average transformation efficiency in four series of experiments (total number of calli - 2299) was found to be 1.84% (ranging from 0.3% to 3.4%), while total co-transformation efficiency reached 87.93%.
Conclusion:
The high efficiency of co-transformation in the experiment promotes it as a very useful technique for the production of wheat lines, free of the selectable marker gene. To our knowledge, this is the first report of cisgenic bread wheat, where both target and selectable genes are derived from wheat.
1. INTRODUCTION
Wheat is one of the main cereal crops of the world. According to the estimations of the Food and Agriculture Organization, the worldwide production of wheat continues to increase, with almost 2 790 million tonnes to be produced in 2020. Kazakhstan is the fourth largest exporter of wheat in the world [1]. The quality and yield of this cereal are influenced by a variety of factors, with fungal diseases being one of the most important. Fungal infection causes a decrease in harvest quantity and quality, with a 30-40% yield loss in some years [2]. Once mycotoxins are detected, wheat cannot be further used for food.
Wheat plants respond to fungal infection by expression of a whole set of pathogenesis-related genes (PR-genes). The most studied and straightforward PR-genes are hydrolytic enzymes, such as chitinase and β-1,3-glucanase. These enzymes hydrolyse molecules of the fungal cell wall (chitin and β-glucan) and also stimulate further response when reacting products serve as signal molecules for the plant’s response [3, 4].
One of the approaches used to increase wheat resistance to fungal infections is the overexpression of the resistance genes [5], including chitinases [6-10]. The most commonly used methods for gene introduction into the plant genome are agrobacterial and biolistic transformation [11]. The first is based on the ability of Agrobacterium tumefaciens to carry a target DNA sequence as a part of its own T-DNA and “upload” it in the host genome. However, this system is constrained by the resistance of plant cells to this pathogen [12]. Since all monocotyledons like wheat are naturally resistant to Agrobacterium infection [13], this method has limited utility. The second method – biolistics –depends more on the regenerative capabilities of plant tissue, allowing a wider variety of species to be targeted for application. Biolistics is a physical delivery approach that has been successfully applied to all the main cereal cultures, including maize, rice, wheat, barley, and sorghum [11]. High-copy inserts of the target sequence in the host genome are the main disadvantage.
When talking about the genetic transformation of cereals and their potential use in the selection of new lines and varieties, we need to account for its potential acceptance (or lack thereof) by the general public [14]. Since transgenic plants (using genes from other species) are not widely accepted by the general public at this time, a new direction of plant genetic engineering is emerging, called cisgenics [15] - the use of genes of the same or closely related species, i.e., from sexually compatible DNA pool. This new approach has been successfully used for breeding disease-resistant plants many times, increasing resistance of potato to late blight (using genes from wild relatives), apples resistance to scab, and strawberry’s resistance to gray mold via overexpression of its own PGIP gene [16, 17].
With wheat in high demand as staple food worldwide, methodical approaches to its biolistic transformation are much needed as an alternative to transgenic “GMO” modification. The goal of this study was to fine-tune methods of biolistic co-transformation of wheat using this cisgenic approach in wheat crop breeding.
2. MATERIALS AND METHODS
2.1. Plant Material and Growth Condition
Spring bread wheat Triticum aestivum cv. Saratovskaya 29 (M.A. Aitkhozhin Institute of Molecular Biology and Biochemistry, Almaty, Kazakhstan) was used for transformation. Plants were grown in the greenhouse with 16-hour light day conditions. Spikes were collected 14 days post-anthesis, and immature caryopses were sterilized by 70% ethanol (v/v) and 0.6% NaOCl with Tween 20, followed by rinsing three times with sterile water. Immature embryos were excised in a laminar flow under a stereoscopic microscope and placed on Murashige and Skoog medium [18] with centrophenoxine (4.43 g/l Murashige and Skoog basal medium with vitamins, 0.5 g/l casein acid hydrolysate, 40 ul/l cupric sulfate, 30 g/l sucrose, 30 mg/l centrophenoxine hydrochloride, 5 g/l Phytagel). Petri plates with excised embryos were kept in the dark at room temperature for 2-3 weeks with embryo-side in contact with the medium. Parts of resulting embryogenic calli were excised from the explants under a stereoscopic microscope and transferred to MS-based osmotic media (4.43 g/l Murashige and Skoog basal medium with vitamins, 0.5 g/l casein acid hydrolysate, 120 g/l sucrose, 40 ul/l cupric sulfate, 5 g/l Phytagel) prior to genetic transformation.
2.2. Genes and Constructs
Class I chitinase gene was cloned in the Laboratory of Genome of M.A. Aitkhozhin Institute of Molecular Biology and Biochemistry. A full-size gene of 957 base pairs (bp) was inserted into a cloning vector by Rapid DNA Ligation Kit and multiplied in dam-/dcm- chemically competent E. coli cells. The presence of the insert was checked by plasmid DNA restriction with the XmnI enzyme. MEU – minimal expression unit – of 3,272 bp, consisting of maize ubiquitin promotor, chitinase gene, and terminator, was excised from the cloning vector by SpeI and XmaI restriction enzymes with its further dephosphorylation by SAP enzyme in accordance with manufacturer’s instructions. The fragment (MEU) was eluted from 1% agarose gel by GenElute Gel Extraction Kit (Sigma-Aldrich).
Minimal expression unit containing Acetohydroxyacid Synthase (AHAS) gene driven by ubiquitin promoter, which provided resistance to imidazolinone herbicides and was used for co-transformation, was courtesy of Australian Center for Plant Functional Genomics.
2.3. Biolistic Transformation and Regeneration
The biolistic transformation was carried out according to the protocol [19] using Biolistic Particle Delivery System PDS-1000/He (Bio-Rad). Embryogenic callus was incubated on osmotic media for not less than 4 hours. The minimal expression units of both chitinase and AHAS (100 ng each) were resuspended in 50 ul of gold particles with coating solution [19].
After particle bombardment, the calli were kept in the dark for 20-24 hours and then recovered on an MS-based recovery media (4.43 g/l Murashige and Skoog basal medium with vitamins, 0.5 g/l casein acid hydrolysate, 60 g/l sucrose, 40 ul/l cupric sulfate, 25 mg/l centrophenoxine hydrochloride, 5 g/l Phytagel). In one week, the calli were subcultured to MS selection media (4.43 g/l Murashige and Skoog basal medium with vitamins, 0.5 g/l casein acid hydrolysate, 30 g/l sucrose, 40 ul/l cupric sulfate, 25 mg/l centrophenoxine hydrochloride, 0.5 uM imazethapyr, 5 g/l Phytagel) and kept in the dark for two months at room temperature for further selection.
Calli that successfully underwent selection were transferred to regeneration medium (4.43 g/l Murashige and Skoog basal medium with vitamins, 0.5 g/l casein acid hydrolysate, 20 g/l maltose, 200 ul/l cupric sulfate, 1 mg/l kinetin, 5 mg/l zeatin, 5 g/l Phytagel) for shoot production and cultured in a 16-hour light period at 22-24°C for 2-4 weeks. Upon induction of shoots with two leaves, the cultures were transferred to rooting medium (2.2 g/l Murashige and Skoog basal medium with vitamins, 0.25 g/l casein acid hydrolysate, 25 ul/l cupric sulfate, 15 g/l sucrose, 0.25 uM imazethapyr, 4 g/l Phytagel) for the second round of selection. The resulting plantlets were transferred to soil and grown to maturity in the greenhouse conditions of 16-hour light period and 22-24°C.
2.4. PCR Identification of Cisgenic Plants
For the identification of cisgenic plants, genomic DNA was extracted from the leaves of imazethapyr-resistant plants at the plantlets stage. Wheat DNA was extracted with Extract-N-Amp Red Plant PCR Kit (Sigma-Aldrich) following manufacturer’s instructions. Genomic DNA of non-transformed plants and water were both used as a negative control, while plasmid DNA was used as a positive control for the experiment. The cisgenic plants carrying chitinase target gene were identified by PCR using forward primer (5- ACCCTGTTGTTTGGTGTTACTTCTGC) and reverse primer (5- GCAGTAGCCCCAGGAGTAGG) with the following program: 96°C for 10 minutes, followed by 45 cycles of 1 min at 95°C, 1 min at 58°C, 1 min at 72°C, and 5 minutes extension at 72°C. The expected product of 529 bp in size was visualized by electrophoresis in 1.5% agarose gel stained by ethidium bromide.
3. RESULTS
3.1. Wheat Donor Material Selection
Preliminary work has been conducted for this study to find Kazakh wheat varieties best suitable for tissue culture, which resulted in the selection of six varieties for further work (data not shown). The variety of spring bread wheat Saratovskaya 29 showed the best qualitative characteristics in the process and was chosen as the main donor material. It is Kazakhstan’s classic original variety used in selection process for acquiring new wheat lines with improved qualities.
The plant material for embryogenic calli, grown in the controlled conditions in the greenhouse, served as a source of immature embryos used as explants (Fig. 1). A thorough selection of the original explant material allows less work at later stages when choosing material for biolistic experiments, saving time and labor used for processing the explants that have less chance to result in embryogenic callus. Therefore, the seeds were checked twice for the suitable developmental stage of the immature embryos: once in the greenhouse before the wheat head was cut and then controlled by a stereoscopic microscope when excising embryos in sterile conditions. Only the embryos with the correct size (2-3 mm) and developmental stage were cultured on the callus-inducing medium.
The same idea of thorough material selection was kept in mind when inducing callus – the time was optimized to achieve the maximum amount of embryogenic callus. One of the conventional approaches to cereal transformation is using calli from immature embryos obtained 2-7 days after callus induction [10, 20, 21]. In our work, we used embryogenic callus from longer cultivation. Partly, this longer period on the callus-inducing medium was caused by variety-specific peculiarities of Saratovskaya 29. On the other hand, the longer cultivation allowed a more precise screening of the material for further manipulations.
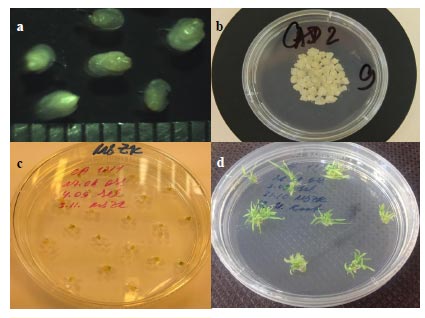
3.2. Biolistic Transformation
The chitinase gene used for the experiment was cloned from the Kazakhstan wheat variety Stepnaya 15 [22]. This variety is characterized by increased resistance to pathogens, hence making it a good source of resistance genes. The sequencing data of the cloned gene was aligned with similar sequences found in the international database to prove it is a Class I chitinase. The analysis of the alignment established 27 changes, 22 of which are synonymous, and 5 lead to changes in the amino acid sequence of the chitinase protein [23].
One of the objectives of the study was to use a cisgenic approach. Thus, the method of gene introduction into the wheat genome – biolistic transformation – was chosen to completely exclude unnecessary DNA sequences by using only Minimal Expression Units (MEU), as opposed to an agrobacterial transformation that might leave traces of the vector itself (T-DNA). Fig. (2) shows the vector used for amplification of the minimal expression unit used in the research.
The promoter chosen for constitutive expression of the target gene in wheat cells was the maize Ubiquitin Promoter (Ubi1), which has a good reputation in the stable transformation of cereals and is actively used for overexpression of target genes in wheat [6, 9, 10, 20].
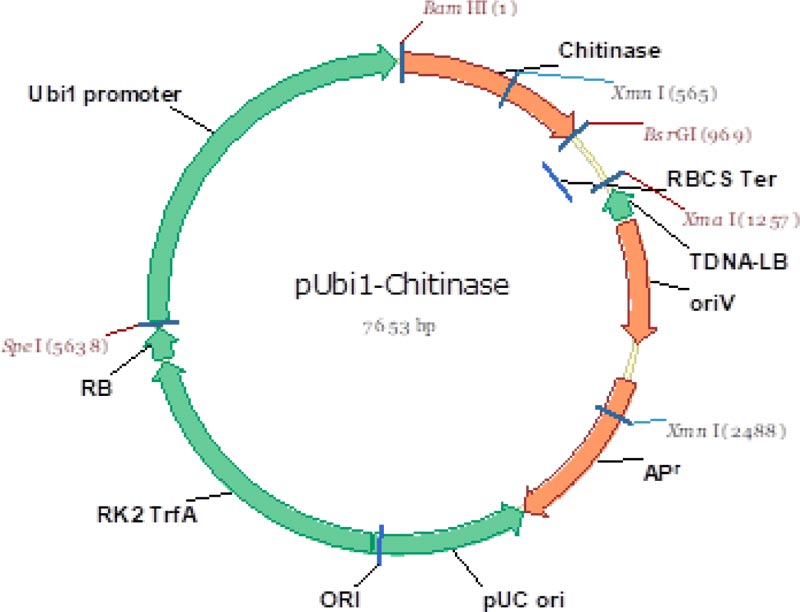
For a further selection of the transformed regenerant plants, the chitinase minimal expression unit was co-transformed with another construct containing Acetohydroxyacid Synthase (AHAS) gene, providing tolerance to the imidazolinone herbicides. This gene is present in wheat and codes for the enzyme acetohydroxyacid synthase, which plays a leading role in the biosynthesis of branched-chain amino acids. There are three copies in wheat’s genome, one in each of A, B, and D genomes [24]. It is also present in fungi, bacteria, and all other plants and serves as a target to imidazolinone herbicides, which fill the substrate-access channel, thus inactivating the enzyme [25, 26]. The co-transformation strategy, instead of using one plasmid containing two genes, proved to be more effective and increases the transformation efficiency [27]. It is noteworthy that the integration is unlinked in this case, making it possible to select the progeny with only one target gene after possible segregation.
The AHAS gene was chosen to make the experiment cisgenic since, most often, selective markers used in wheat transformation are initially cloned from other organisms. One of the most popular genes used for selection is bar, conferring resistance to herbicide bialaphos [28]. Many studies involving resistance genes’ introduction into the genome of wheat used this gene [6, 9, 10, 29, 30]. Cisgenic approach to wheat transformation was used previously in the improvement of wheat baking qualities, and even in that case, only the target gene was cisgenic, while the selection marker – gene pmi – was bacterial [21].
3.3. Analysis of Regenerated Plants
The regenerant plants growing on selective media with herbicide imazethapyr and potentially carrying AHAS gene were checked for its presence. The PCR analysis showed that 58 out of 59 plants have the necessary band (524 bp, Fig. (3)), meaning that one plant was herbicide-tolerant due to other mechanisms.
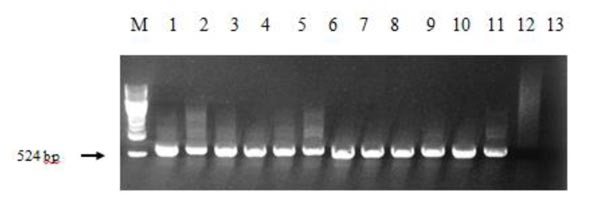
The regenerant plants were also checked for the presence of chitinase construct. When designing primers for analysis of the introduced target gene in cisgenic works, one should account for the genes with the same or similar nucleotide sequence in the object's genome. In this case, the chitinase genes are present in the wheat genome, so the specific primers were designed to amplify the area at the border of the Ubi1 promoter and chitinase coding sequence. Fig. (4) presents the PCR results; plasmid DNA with minimal expression unit served as a positive control.
As shown in the Fig. (4), the chitinase insert-positive plants have the necessary amplicon of 529 bp. The experimental plant in lane 8 does not have the needed band, meaning that this plant is free of the chitinase construct. The results of PCR analysis proved that 51 plants of T.aestivum cv. Saratovskaya 29 were successfully transformed with class I wheat chitinase.
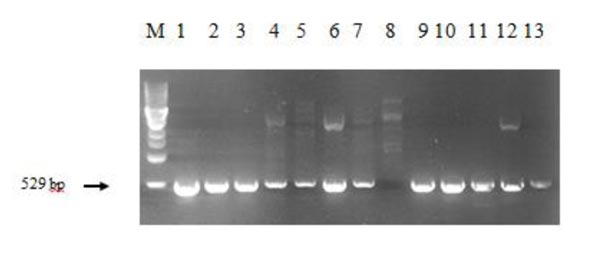
Thus, 51 plants out of 59 regenerants were co-transformed with two genes, seven plants have only AHAS gene insert, and one plant is free of target sequences and probably escaped the selection due to other tolerance mechanisms or factors.
3.4. Transformation efficiency
The given study involved four series of experiments with a total number of 2299 calli. The results of transformation efficiency for each experimental series are given in Table 1.
Experiment series | Number of embryos (calli) | Number of cisgenic plants | Transformation efficiency, % |
---|---|---|---|
1 | 703 | 24 | 3.4 |
2 | 488 | 4 | 0.82 |
3 | 336 | 1 | 0.3 |
4 | 772 | 22 | 2.85 |
Average transformation efficiency was found to be 1.84%, with a maximum of 3.4% in the first series of experiments and a minimum of 0.3% in the third series.
Total co-transformation efficiency – 51 out of 58 plants carrying both of the inserts at the same time – reached 87.93%.
4. DISCUSSION
The effectiveness of genetically modified crops has been well documented, revealing its positive influence on crop yield, farmers’ benefits while decreasing pesticide use, especially in developing countries [31]. As there are public concerns over genetically modified plants (transgenic plants), a cisgenic approach was introduced, which should be more accepted by the general audience [32-34, 14].To date, there are not many reports of cisgenic crops, with cereals represented by barley [35] and durum wheat [21]. To our knowledge, this is the first report of cisgenic bread wheat, where both target and selective genes are derived from wheat.
The approach used for embryogenic callus production – longer cultivation before actual biolistic transformation – is novel and, to some extent, contradicts the conventional method of taking very fresh, maximum one week-grown immature embryos into the experiment. At the same time, it let us increase the mass of embryogenic callus because of its growth, making it easier to differentiate embryogenic callus from the other tissue types. Usually, the regeneration abilities of the donor material decrease quite rapidly, leading to poorly regenerating cultures after 2-3 weeks of cultivation on callus induction media. We also need to account for variety-specific peculiarities, in this case, Saratovskaya 29, leading to better production of embryogenic callus at a later stage. It is yet another evidence that stable wheat transformation requires identification of the donor varieties, which not only possess valuable traits but are at the same time “transformation-friendly.” This implies better characteristics in the tissue culture, starting from embryogenic callus production to whole plant regeneration.
An overview of the successful biolistic experiments [27] shows a very wide range of transformation efficiency starting from 0.1% and up to 70% (a 90% efficiency mentioned in the article is actually the effectiveness of plants' regeneration after the bombardment). In half of these works (11 out of 22), the efficiency does not exceed 1. It should be noted further that most of the experiments were done on bread wheat variety Bobwhite, which was chosen as a model for these studies because of its outstanding performance in tissue culture. The average transformation efficiency of 1.84%, reported in this study, is in line with the best results of other varieties worldwide, making Saratovskaya 29 the first-choice variety for further cisgenic improvement in a very wide region, including Russia and Kazakhstan, where it is produced.
One of the limitations of the biolistic method is low transformation efficiency. It depends on the cells' quantity and genotype, their regeneration capability, quantity of DNA-coated metal particles, quantity and quality of the DNA construct, physical parameters of the bombardment, and other factors, and constitutes about 0.002-0.01% of transformation efficiency [20, 21]. The efficiency of co-transformation in the experiment was high (87.93%), suggesting it to be a very useful technique for the production of wheat lines, free of the selectable marker gene, by selecting plants with segregated AHAS insert in the next generation. The material for all biolistic experiments was chosen among induced callus using the same criteria, and the biolistic procedure was carried out following the same protocol. So, the variability of the transformation efficiency in different experimental series is probably caused by the differences in the genotype of the used callus cells. This supports the prevailing opinion that the key drawback of wheat transformation is genotype dependency [30]. Nevertheless, our results show higher transformation efficiency than the average and show the perspectives of the given approach in improving spring bread wheat qualities.
The findings of this study might be used in the production of transformed wheat plants. The plants obtained in the course of the study carrying wheat class I chitinase gene will be tested for resistance/tolerance to fungal pathogens and, if successful, will hopefully be used in crop breeding for improved wheat.
CONCLUSION
While genetic transformation is widely used among cultivated plants, wheat is not as easily transformed as some other crops due to the low efficiency and genotype dependency [27]. At the same time, there is increasing demand for the production of new varieties with improved characteristics, such as pathogen resistance. So, the studies on the improvement of major crop cultures such as the one provided in this article are of great importance for promoting world food security.
ETHICS APPROVAL AND CONSENT TO PARTICIPATE
Not applicable.
HUMAN AND ANIMAL RIGHTS
Not applicable.
CONSENT FOR PUBLICATION
Not applicable.
AVAILABILITY OF DATA AND MATERIALS
Not applicable.
FUNDING
This work was part of the state-funded Ph.D. program. It was also supported by AP05136045, “New lines of wheat and potato created using a complex of innovational approaches of plant genetic engineering and cell technologies” funded by the Ministry of Education and Sciences of the Republic of Kazakhstan.
CONFLICT OF INTEREST
The authors declare no conflict of interest, financial or otherwise.
ACKNOWLEDGEMENTS
The authors acknowledge the Ministry of Education and Sciences of the Republic of Kazakhstan for funding this research.