All published articles of this journal are available on ScienceDirect.
Enhanced Reusability of Horseradish Peroxidase Immobilized onto Graphene Oxide/Magnetic Chitosan Beads for Cost Effective Cholesterol Oxidase Assay
Abstract
Background:
Horseradish Peroxidase (HRP) is an important biocatalyst extensively used in enzymatic reactions. Cholesterol oxidase (ChoX) is a commercially valuable enzyme used in the estimation of cholesterol in human serum. ChoX is an oxygen oxidoreductase class of enzyme which catalyzes the oxidation of cholesterol in the presence of O2, liberating hydrogen peroxide H2O2 as a by-product. HRP catalyzes the reduction of this H2O2 in the presence of a redox dye (chromophore), producing a pink colored Quinoneimine which can be measured spectrophotometrically. The use of soluble HRP makes this assay method expensive for each time use and the recovery of HRP is not possible.
Objective:
Our aim was to prepare the HRP immobilized beads having magnetic properties for the ease of separation and increasing the reusability of HRP for the low cost ChoX assay.
Methods:
In the present work, we prepared magnetic chitosan beads using chitosan-Fe2O3 nanoparticle blend coated with Graphene Oxide (GO), and subsequently activated with 2.5% glutaraldehyde (GA). Enzyme loaded beads were characterized by SEM, FTIR, and XRD analysis.
Results:
The immobilization efficiency was ~80% and the immobilized HRP retained 90% of its initial activity up to 12 times reuse. The pH and temperature optima were shifted from 6.5 and 50°C for soluble HRP to 7.0 and 55°C for the immobilized HRP, respectively. Storage stability of immobilized HRP was 93.72% and 60.97% after 30 and 60 days storage respectively, at 4°C.
Conclusion:
On the basis of the present study, the HRP loaded magnetic chitosan/graphene oxide beads could be used for low-cost ChoX assay at laboratory scale due to its enhanced reusability and stability.
1. INTRODUCTION
Enzymes, as biocatalysts, have been enormously used in several industries such as food, pharmaceuticals [1, 2], textiles [3], etc. Apart from these, enzymes are exclusively preferred for various biotechnological applications such as immunosensors used in clinical diagnostics [4], biotransformation reactions [5] and the enzymatic endpoint method based assay of biochemicals in bioanalytical chemistry [6] because of this pronoun is used for enzyme indicating its functonality high substrate specificity and catalytic activity. Many enzymes are well known for their applications in biosensors and clinical chemistry viz. glucose oxidase [7, 8], cholesterol oxidase [9], horseradish peroxidase [10], urease [11], etc. The biochemical reactions catalyzed by these enzymes play a key role in the estimation of glucose, cholesterol, hydrogen peroxide and urea which are highly specific, selective and have no chemical substitute for the reactions they catalyze. On one hand, the enzyme-catalyzed reactions due to their high specificity, selectivity no use of corrosive reagents, extreme pH and temperature ranges gain an advantage over the conventional chemical catalysts, at the same time, they suffer from the shortcomings of high cost and low stability.
In the past decade, the use of enzymes in diagnostic kits and development of biosensors have increased their commercial value to attend to new market needs. The use of specific reaction conditions and their non-reusability in the reaction mixture adds to the cost of enzymatic assays they catalyze. Moreover, their possible inactivation caused by various physical and chemical agents viz. pH, temperature, organic solvents, detergents and salts poses a question on operational stability and storage stability of these enzymes. Enzyme immobilization technique has emerged as a possible solution to overcome the problems pertaining to the enhancement of catalytic activity and stability, while the recovery and reusability of biocatalyst reduce the overall cost. Immobilization of soluble enzymes to a suitable support matrix is the most influential and efficient means to optimize the specific properties of enzymes (catalytic activity, stability, and specificity), as well as minimize the enzyme inhibition effects, and aids in the recovery and reuse of the biocatalyst. In addition, the use of immobilization technology increases the industrial importance of the biocatalyst and is therefore highly significant from an economic point of view [12, 13].
Horseradish peroxidase (HRP, E.C.1.11.1.7) has been widely used and investigated for analytical purposes [10]. Hydrogen peroxide (H2O2) is liberated as a by-product in almost all the biochemical assays catalyzed by oxidases class of enzymes. HRP catalyzes the reduction of H2O2 in the presence of a reducing agent (chromogen) to produce a colored adduct. For the indirect estimation of oxidase substrates (e.g., Glucose, Cholesterol) HRP is coupled with H2O2 produced by the oxidases (e.g., Glucose oxidase or Cholesterol oxidase). The use of soluble HRP in the estimation of cholesterol oxidase by enzymatic endpoint method makes this assay method expensive due to its poor reusability and low stability. Enzyme immobilization technology offers a straightforward and most effective solution to reduce the cost of this biochemical assay by increasing the reusability and storage stability of soluble HRP.
Covalent binding technique is usually preferred over the physical adsorption method for the immobilization of enzymes as it essentially prevents enzyme leaching from the carrier surface, thereby increasing its reusability and longevity in storage conditions [14]. The use of Magnetic Nanoparticles (MNPs) for enzyme immobilization imparts easy separation property to the biocatalyst from the reaction medium; however, the available literatures suggest that most of the enzymes immobilized on MNPs were physically adsorbed leading to weak interactions between the carrier and the enzyme. The conjugation of MNPs with a biopolymer such as chitosan protects the Nanoparticles (NPs) from erosion and provides promising functional groups for enzyme attachment. Chitosan is a polysaccharide, characterized with its unique biochemical properties including biocompatibility, bioactivity, biodegradability, non-toxicity, and functionalized groups, making it an excellent biopolymer for both coating the MNPs and enzyme immobilization [15]. The free hydroxyl and amino groups of chitosan encompasses the enzyme attachment by both adsorption and covalent linkage mechanism. Graphene Oxide (GO) is an amphiphilic macromolecule with a hydrophobic plane and hydrophilic edges [16]. It contains multiple functional groups including epoxy, hydroxyl, carboxyl and carbonyl groups on its surface due to which it is easily dispersed in water as a colloidal suspension [17]. These functional groups on its surface are readily available to interact with the enzymes thus making it an ideal support for enzyme immobilization. GO also enhances the integrity of the synthesized biocatalyst by providing high mechanical strength. Covalent immobilization of the enzyme on the support surface by using GA as a crosslinking agent increases the enzyme attachment, thereby increasing the immobilization efficiency. GA is readily available at a relatively low cost and is highly reactive with the amine groups at around neutral pH, due to which it imparts efficient and effective crosslinking as compared to other aldehydes.
In the present work, we prepared GO coated Fe2O3/ chitosan beads, used as a carrier support for HRP immobilization by covalent crosslinking with GA treatment. We were further interested in the application of these GO coated magnetic chitosan beads for increasing the reusability of HRP in ChoX assay. To the best of our knowledge, we used this hybrid carrier support for HRP immobilization and as far as we know, till now, there have not been any reports concerned with such strategy. Some optimal operational conditions for immobilized HRP were established and the stability of immobilized HRP under various conditions was studied.
2. MATERIALS AND METHODS
2.1. Chemicals Used
Cholesterol was purchased from Sigma Aldrich Pvt. Ltd. Chitosan (deacetylation degrees higher than 85%), Horseradish Peroxidase and single layer Graphene oxide nanopowder were purchased from the Sisco Research Laboratories, Mumbai, India. All other chemicals used were of analytical grade and used without purification.
2.2. Preparation of Magnetic Nanoparticles
Magnetic iron oxide nanoparticle was prepared by the thermal co-precipitation method described by Moghaddam and Aliahmad with slight modifications [18]. The synthesis of maghemite NPs was carried out using a mixture of aqueous solutions of Fe2Cl3 (100 mL, 1 M) and FeSO4 (100 mL, 0.5 M). The above mixture was heated up to 90°C along with the addition of NH4OH (25% v/v of ammonia) dropwise, with vigorous stirring till it attains the alkaline condition (pH 10.0). The color of the mixture slowly turned to black while reaching the alkaline pH. The mixture was continuously stirred for at least 30 min and centrifuged at 5,000 rpm for 5 min. The black precipitate obtained was washed with deionised water till neutral pH of the supernatant was obtained, dried in a hot air oven at 60°C and crushed using a pestle and mortar. The black powder so obtained was Fe3O4 NPs (magnetite). For the synthesis of Fe2O3 (maghemite) NPs, the black powder was kept at 200°C in a hot air oven for 14-16 h till it turned to a reddish-brown color. This powder was cooled to room temperature and crushed again by a pestle and mortar.
2.2. Preparation of Magnetic Chitosan Beads
Chitosan was dissolved in acetic acid (25 mL, 2% v/v) with the help of a magnetic stirrer overnight, to assure no lump formation, until a clear transparent solution was obtained. The trapped air bubbles were removed by sonicating the chitosan solution for 20 min. Fe2O3 NPs prepared in section 2.2 were treated with ethanol in order to remove any moisture in it and air dried. Fe2O3 (2 g) was added slowly to the chitosan solution and vigorously stirred for 1 h till the NPs were homogeneously dispersed in the chitosan solution to form a blend. The chitosan-Fe2O3 blend was used for preparing chitosan beads having magnetic properties with the help of a 5 mL syringe, in NaOH (100 mL, 1 M) under continuous stirring. The beads were left in the same NaOH solution for 4 h for hardening and then washed with deionised water until neutral pH of the washings was obtained.
2.3. Coating Graphene Oxide on Magnetic Chitosan Beads
After extensive washing with deionised water, the Magnetic Chitosan (MC) beads (100 mg/mL) were transferred to an exfoliated and homogeneous solution of GO (stock solution 0.4 mg/mL) and was stirred overnight. The MC beads were separated from the GO solution using an external magnet. The GO solution can be reused for the second cycle of GO coating on MC beads. The MC beads were extensively washed for the removal of excess GO from beads surface. The GO coated MC beads (GOMC beads) were sonicated for 1 h in a bath sonicator, washed with deionised water and lyophilized for 6-8 h.
2.4. Immobilization of HRP on GO Coated Magnetic Chitosan Beads
The activation and functionalization of GOMC beads were carried out by treatment with GA (2.5% v/v) at 25°C for 8 h. The aldehyde functionalized GOMC beads were separated using an external magnet and washed at least five times to remove any unreacted GA residues. The obtained GA activated GOMC beads were stored in potassium phosphate buffer (50mM, pH 7.0).
HRP was covalently immobilized on the surface of GOMC beads by the GA activation method [19]. The GA-activated GOMC beads were immersed in a cocktail solution of HRP (10mg/100 beads) and phosphate buffer (50mM, pH 7.0) followed by overnight incubation at 30°C, 200 rpm in an orbital shaker (Orbitek, Scigenics Biotech Pvt. Ltd., Chennai, India) for immobilization. After completing the immobilization procedure, the peroxidase immobilized Graphene Oxide coated Magnetic Chitosan beads (PGOMC) were separated using an external magnet. The PGOMC beads were washed four to five times with phosphate buffer (50mM, pH 7.0) to remove the unbound peroxidase till no protein was detected in the washings. The PGOMC beads were preserved in phosphate buffer (50mM, pH 7.0) in a refrigerator at 4°C for further studies and lyophilized (6-8 h) before structural characterization.
The protein concentration was determined spectrophotometrically according to the method described by Bradford [20]. Bovine serum albumin was used as a standard protein for the construction of a calibration curve. The amount of immobilized protein was evaluated by calculating the difference between the initial protein concentrations of the prepared enzyme solution subjected for immobilization and the residual protein left in the same enzyme solution after immobilization.
![]() |
(1) |
2.5. Characterization of the HRP Loaded Magnetic Beads
Surface morphology of the synthesized beads was analysed with a Zeiss EVO 18 scanning electron microscope (Zeiss, Germany) using 20 kV accelerating voltage. X-Ray Diffraction (XRD) spectrum of the synthesized material was obtained for the crystallization phase analysis (Rigaku diffractometer). The measurement was carried out in the Bragg’s angle (2θ) ranging from 10°-80°, at a scanning rate of 5° min−1, Cu Kα radiation (λ = 0.15418 nm) using Rigaku Miniflex 600 D Tex ultra. Magnetization curve of the synthesized magnetic nanoparticle was obtained using a Vibration Sample Magnetometer (VSM, Riken Denshi Co. Ltd., Japan) at room temperature. The analysis of the functional groups attached to the carrier support and the enzyme was performed using Fourier Transform Infrared Spectroscopy (FTIR). The samples were scanned in the range of 4000-400 cm-1 using FTIR spectrophotometer (Shimadzu, Japan).
2.6. Activity Assay of Free and Immobilized Enzyme
The estimation of peroxidase activity was done by coupling H2O2 with 4-aminoantipyrine and phenol in the presence of peroxidase to form quinoneimine; a chromogen having an intense pink color with the absorption maxima at 510 nm. The amount of quinoneimine produced was directly proportional to the amount of peroxidase present [21]. 1 mL of the reaction mixture consisted of 0.05 M potassium phosphate buffer at pH 7.0, 0.0025 M 4-aminoantipyrine with 0.17 M phenol and soluble or immobilized HRP, pre-incubated for 3 min at 30°C. The reaction was initiated by adding 0.0017 M H2O2 to the pre-incubated reaction mixture and the incubation continued for 10 min at 30°C. The reaction was terminated by heating the samples in a boiling water bath for 2 min followed by subsequently transferring the samples into an ice-bath for color development. The PGOMC beads were magnetically separated before the termination of the reaction. Blank was prepared by taking the reaction mixture containing all the components excluding HRP (free or immobilized). Absorbance was recorded at 510 nm by the discontinuous spectrophotometric method (UV 1800 Spectrophotometer, Shimadzu, Japan). One unit of activity was defined as the amount of HRP required to hydrolyze one micromole of H2O2 per minute at 30°C and pH 7.0.
![]() |
(2) |
2.7. Application of PGOMC Beads for Cholesterol Oxidase Assay
The prepared PGOMC beads were examined for their efficacy to estimate ChoX in the reaction mixture containing cholesterol as a substrate by the modified method of Allain et al. [22]. The reaction conditions for the ChoX assay were optimized in our previous study [23]. Cholesterol (50 µL; 6 g/L) dissolved in Triton X-100 5% (v/v) and dimethylformamide was added to 1 mL of the reaction mixture containing 1mM 4-aminoantipyrine, 5 mM phenol, and sodium phosphate buffer (20 mM, pH 7.0). The prepared PGOMC beads (mentioned in section 2.5) loaded with 10 U HRP (4 beads) were added to the above reaction mixture and pre-incubated for 5 min at 30°C. 100 µL of crude ChoX extracted from the supernatant of the fermentation broth of Streptomyces olivaceus MTCC 6820 was added to the pre-incubated reaction mixture to start the reaction and the incubation continued for 10 min at 30°C. The PGOMC beads were separated by an external magnet before the termination of the reaction. The reaction was terminated by placing the samples in a boiling water bath for 2 min and then immediately placed in an ice bath for 2 min for color development. Absorbance was recorded at 500 nm (UV 1800 Spectrophotometer, Shimadzu, Japan). Blank was prepared by adding an inactivated enzyme to the reaction mixture. One unit of ChoX activity was defined as the amount of enzyme that converts 1µmol of cholesterol into H2O2 per minute at 30°C.
The use of immobilized HRP in the enzymatic assay method of ChoX can be explained by the following biochemical reaction. The oxidation of cholesterol is catalyzed by the ChoX enzyme to produce H2O2; the degradation of H2O2 is further catalyzed by HRP to produce quinoneimine dye having absorption maxima at 510 nm.
![]() |
(3) |
2.8. Properties of Immobilized HRP
2.8.1. Effect of pH
The study of optimal pH for both the free and immobilized HRP was conducted by measuring the enzyme activity over the pH range of 4 to 9 using the following buffers (0.05 M): sodium acetate buffer (pH 4.0 to 5.5), potassium phosphate buffer (pH 6.0 to 7.5), and Tris-HCl buffer (pH 8.0 to 9.0). Blank was prepared using potassium phosphate buffer pH 7.0. Free and immobilized HRP along with respective pH buffers were incubated for 10 min at 30°C and the enzyme activities were measured as mentioned in section 2.6.
2.8.2. Effect of Temperature
The study of optimal temperature was carried out by measuring the enzyme activities in the temperature range of 25 to 85°C. Both the free and immobilized HRP were incubated for 10 min at different temperatures along with the reaction mixture using potassium phosphate buffer (0.05M, pH 7.0), and enzyme activities were measured as mentioned in section 2.6.
2.8.3. Thermostability
The thermal stability studies were carried out at three different temperatures viz. 35°C, 55°C and 75°C. Both the free and immobilized enzymes were incubated using phosphate buffer (0.05 M, pH 7.0) at the respective temperatures for 2 h. 100 µl samples from each temperature variable were tested for peroxidase activity using phosphate buffer pH 7.0 (refer section 2.6).
2.8.4. Effect of Metal Ions
The effect of metal ions on both free and immobilized HRP was studied in the presence of chloride and sulphate salts of different metal ions viz. NaCl, KCl, MgCl2, CaCl2, HgCl2, PbCl2, ZnCl2, CdCl2, MnCl2, NiCl2, CuSO4, CsCl2. The enzyme was incubated with a 2 mM solution of different metal ions for 15 min and then the enzyme activity assay was performed for both the free and immobilized HRP. The enzyme activity in the absence of metal ions was recorded as 100%.
2.8.5. Stability in Various Detergents and Organic Solvents
Free and immobilized HRP (2.5 U) were incubated overnight with 50% (v/v) suspension of organic solvents viz., isopropanol, ethanol, methanol, and acetonitrile and non-ionic detergents triton X-100, Tween-20, and Tween-80 at 30°C. Samples from each test tube were tested for enzyme activity as mentioned in section 2.6. The enzyme activity assayed in the absence of these compounds was considered as control (100%) for the calculation of residual activity.
2.8.6. Reusability and Storage Stability of Immobilized Enzyme
The activity assay for ChoX using PGOMC beads was performed for 10 consecutive cycles according to the method mentioned in section 2.7. After the completion of each cycle, the same PGOMC beads were separated magnetically, washed with phosphate buffer (50 mM, pH 7.0) and reused in the new reaction cycle without any treatment. The activity of freshly prepared PGOMC beads was taken as 100%. The storage stability of immobilized HRP was monitored by storing the PGOMC beads in a refrigerator for 90 days at 4°C. The activity of PGOMC beads was checked at an interval of every 10 days.
3. RESULTS AND DISCUSSION
3.1. Immobilization
In the present study, we prepared GO coated magnetic chitosan beads for HRP immobilization and characterized the immobilized enzyme. The magnetic chitosan beads were prepared, coated with GO and activated with GA. The immobilization of HRP was successful with nearly 80 ± 1.35% immobilization efficiency. The maximum amount of bound protein was 0.08 mg/bead. The immobilized enzyme
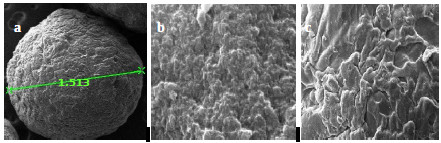
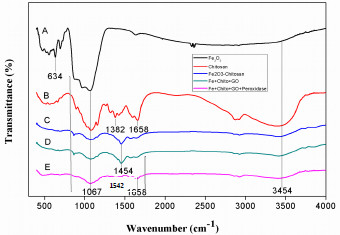
was GA for optimum functional range and stability. GA acted as a cross-linking agent and the activation of chitosan beads by GA generated multipoint attachment sites for the enzyme. Literature suggests that the conjugate of Fe2O3-GO mimics peroxidase-like activity, which may be the reason to enhance the HRP activity [24]. Due to the mimicking of Fe2O3-GO support matrix as peroxidase-like activity, it showed a positive synergistic effect over HRP immobilization than any other support matrix. Moreover, coating GO on the magnetic chitosan beads with sonication allowed the small-sized GO to well-disperse onto the chitosan beads surface, providing uniformity in active sites throughout the beads. With the high immobilization efficiency of ~80%, the GO coated magnetic chitosan beads proved to be an excellent carrier support for HRP immobilization.
3.2. Characterization of Immobilized HRP with Carrier Support
3.2.1. SEM
The SEM image of the HRP immobilized GO coated magnetic chitosan bead is displayed in Fig. (1a, b and c). Spherical beads of an average size of 1.5 mm were formed (Fig. 1a). The SEM image gives an idea about the surface morphology of the PGOMC bead, which indicates the non-porous structure of the bead surface (Fig. 1b). The compactness in the surface morphology may be attributed to the GO coated over the surface of the bead thus increasing the mechanical integrity relatively (Fig. 1c).
3.2.2. FT-IR Analysis
The beads were analyzed by FTIR spectroscopy to determine the interactions between Fe2O3, Chitosan, Fe2O3-Chitosan, Fe2O3-Chitosan-GO, and Fe2O3-Chitosan-GO-HRP, presented in Fig. (2). The peak at 552 cm-1 indicates the presence of iron oxide NPs while the sharp peak at 634 cm-1 corresponds to the characteristic Fe-O vibrational mode of iron oxide NPs (Fig. 2a) [25]. The FTIR spectra of chitosan show troughs at 1382 cm-1 and 1658 cm-1 which are due to the N-H bending vibrations and –C=O stretching of the amide-I group, respectively (Fig. 2b) [26, 27]. The band at 1067 cm-1 corresponds to the C-O stretching vibration due to the presence of saccharide structure in chitosan [28]. It was accounted in the previous studies that the bands appearing in the region of 960 cm-1 to 1180 cm-1 corresponded to the saccharide peaks of chitosan and were consistently maintained throughout (Fig. 1a, b, c and d). The new band present at 1454 cm-1 in the FTIR spectrum of the Fe2O3-Chitosan shows the C-O stretching of the primary alcoholic group (Fig. 2c). The Fe2O3-Chitosan spectrum clearly depicts the presence of all characteristic bands of chitosan. This signifies that Fe2O3 NPs were completely covered with chitosan which confirmed the formation of Fe2O3-Chitosan nano-composite [29]. The band at 1067 cm-1 and 1454 cm-1 is constantly maintained as shown in Fig. (2d) which represents the C-O-C groups present in GO. The new bands observed at 1658 cm-1 and 1542 cm-1 attributed to the –CONH– (amide I) and amide II vibrations Fig. (2e) representing the characteristic transmittance of HRP [30]. These results ascertain the immobilization of HRP on the surface of GOMC beads.
3.2.3. X-Ray Diffraction
The XRD patterns of Fe2O3, Fe2O3-Chitosan, and immobilized HRP are shown in Fig. (3). For Fe2O3 NPs, the peaks at 2θ = 30.1°, 35.4°, 43.1°, 53.2°, 56.9°, and 62.5° correspond to the (220), (311), (400), (422), (511), and (440) planes of Fe2O3, respectively. After coating with chitosan and the immobilization of HRP, the same characteristic peaks revealed that the MNPs uphold the crystalline structure of Fe2O3.
3.2.4. Vibrating Sample Magnetometer (VSM) Measurements
A Vibrating Sample Magnetometer (VSM) was used to measure the magnetic property of the Fe2O3 NPs. The magnetization curve of the Fe2O3 NPs at room temperature is displayed in Fig. (4). The value of the saturation magnetization (Ms) of Fe2O3 was found to be 19 Am2/kg. The synthesized Fe2O3 sample showed super-paramagnetic behavior with 0.015 T coercivity and 0.0018 Am2/kg Remanence is a measure of magnetic induction or magnetic flux density which remains in the magnet after successful magnetization values. Fig. (4) displays a fine hysteresis loop which depends on the size of the NPs. With a decrease in the particle size, there is a decrease in the magnetic domain of each nano particle. The MNPs exist in a single domain below a critical diameter; in this condition, the behavior of the particles is super-paramagnetic. The super-paramagnetic NPs experience fluctuations due to thermal energy which tend to randomize the moments of NPs in the absence of an applied magnetic field; resulting in negligible coercivity and remanence [18, 31]. This indicates the fine crystallite size of γ-Fe2O3 NPs in the range of 20 - 50 nm [32].
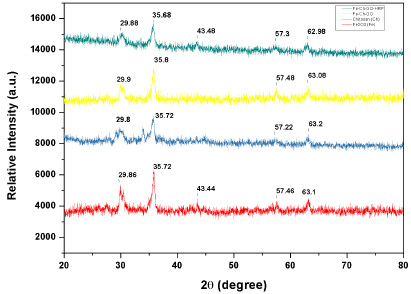
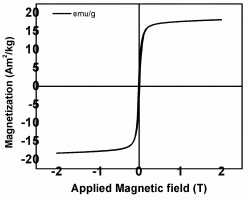
3.3. Properties of Immobilized HRP
Enzymes often exhibit altered kinetic behavior after immobilization which may be attributed to the changes in the microenvironment of the immobilized enzyme provided by the support matrix.
3.3.1. Effect of pH and Temperature on Free and Immobilized HRP
The effect of pH on the activity of free and immobilized HRP was studied by incubating with different buffers in the pH range 4 - 9 at 30°C and the results are presented in Fig. (5a). The pH profile of the immobilized HRP showed that it was mostly active between pH 6-8. However, the optimum pH values for the activity of free and immobilized HRP were 6.5 and 7.0, respectively. Similar results were found, where the pH 6.5 for free HRP shifted to 7.0 for immobilized HRP [33]. A possible reason for this pH shift may be attributed to the conformational changes in the three-dimensional structure of the enzyme protein due to the surface properties of the carrier support after immobilization. The outer surface of the PGOMC bead is rich in free amino groups due to the presence of chitosan, which absorbs H+ ions from the reaction mixture resulting in lower pH value in the vicinity of the immobilized enzyme. In order to account for the conformational change brought about by the immobilization, the pH is raised to a certain degree, facilitating the enzyme to function properly [30]. This shift in the pH optima of the immobilized HRP shows its adaptability towards the environmental alkalinity, which is favorable for its application in the ChoX assay [23].
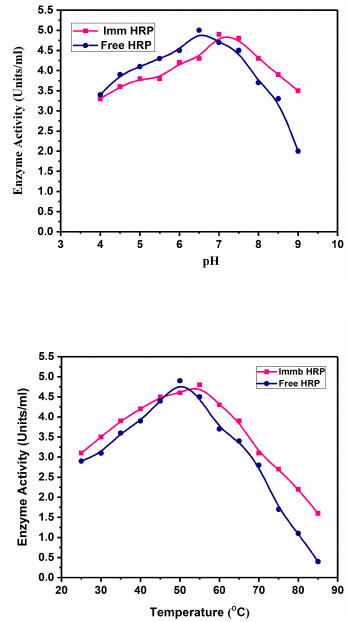
The optimum temperature of the free and immobilized HRP was determined by measuring the enzyme activity at a variable temperature ranging from 25°C to 85°C at pH 7.0 (Fig. 5b). The immobilized enzyme was active over a wide range of temperature viz. 35°C - 65°C. An elevation in the optimum reaction temperature of the free HRP (50°C), as compared to the immobilized HRP (55°C), was observed which was in accordance with the reported literature [34]. This increased heat resistance was possibly because of the support matrix providing a higher degree of cross-linking sites for the attachment of enzyme protein in their active orientation, which shields the HRP from inappropriate structural changes at high temperature. It is well established that if subjected to thermal exposure, enzymes in the soluble form are more prone to the unfolding of protein native three-dimensional conformation thus affecting the protein structure-function relationship which is minimized in case of immobilized enzymes [35].
3.3.2. Thermostability
The thermal stability of free and immobilized HRP was studied at three different temperatures i.e. 35°C, 55°C and 75°C for 2 h. The immobilized HRP maintained 80% activity for 90 min at 35°C, retained more than 70% activity for 90 min at 55°C, and 50% activity up to 60 min at 75°C; Fig. (6a). On the other hand, free HRP retained more than 75% relative activity for 60 min at 35°C, around 45% and 20% of the relative activity up to 30 min at 55°C and 75°C respectively; Fig. (6b). The activity of the enzyme at pH 7.0, 30°C assayed for 10 min was considered as 100% for the calculation of relative activity. An improvement in the thermal stability of the PGOMC beads was observed which was attributed to the covalent attachment of enzyme groups with the free amino ends of chitosan and oxides of GO. The working temperature of the immobilized HRP was widened with respect to time which showed that the GOMC was a good support matrix for electrode preparation using immobilized HRP thereby increasing its stability and applicability in electrochemical biosensors.
3.4. Effect of Detergents, Organic Solvents and Metal Ions
ChoX assay essentially requires the use of various non-ionic detergents and organic solvents for the dissolution of cholesterol (water-insoluble) in the reaction mixture. Therefore, the stability studies of enzymes in the presence of organic solvents and detergents are very important. The activity of free and the immobilized HRP was determined in the presence of ethanol, methanol, isopropanol, acetonitrile, Triton X-100, Tween - 20 and Tween - 80. The results are illustrated in Tables 1 and 2. The free and the immobilized HRP showed a very similar behavioral pattern for the organic solvents and detergents, however, the immobilized HRP exhibited more stability in the presence of these chemicals. The relative activity of the immobilized HRP increased in the presence of methanol, ethanol and isopropanol, whereas it reduced in the presence of acetonitrile. Triton X-100 and Tween-20 enhanced the relative activity of both the free and immobilized HRP. The significant stability of the immobilized HRP in ethanol, Triton X-100 and Tween-20 with relative activity ≥ 150% proved that the PGOMC beads were highly suited for assaying ChoX, as they are the most commonly used solvents in the ChoX assay.
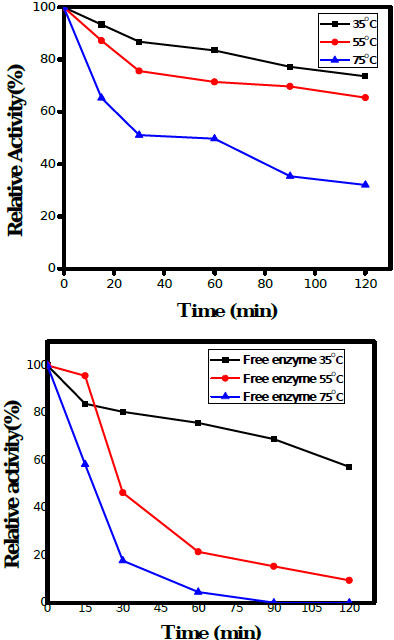
Chemicals | Relative Activity (%) | |
---|---|---|
Immobilized HRP | Soluble HRP | |
Control | 100 | 100 |
Isopropanol | 107.79 | 35.63 |
Ethanol | 155.27 | 57.33 |
Methanol | 100.41 | 33.19 |
Acetonitrile | 72.08 | 23.83 |
Triton X - 100 | 167.82 | 69.9 |
Tween - 20 | 142.5 | 55.24 |
Tween - 80 | 100.5 | 33.8 |
Metal Ions | Relative Activity (%) | |
---|---|---|
Soluble HRP | Immobilized HRP | |
Control | 100 | 100 |
NaCl | 116.19 | 118 |
KCl | 110.5 | 115.62 |
MgCl2 | 98.45 | 177.78 |
CaCl2 | 93.22 | 173.20 |
HgCl2 | - | - |
PbCl2 | 60 | 75.00 |
ZnCl2 | 23.77 | 145.56 |
CdCl2 | 178.12 | 187.25 |
MnCl2 | 84.65 | 198.54 |
NiCl2 | 132.11 | 137.5 |
CuCl2 | - | - |
CsCl2 | 37.30 | 30.27 |
The effects of different metals on free HRP and HRP immobilized on GOMC beads were studied and summarized in Table 2. Mg2+, Ca2+, Zn2+, and Mn2+ showed strong activation effects on immobilized HRP. Zn2+ strongly inhibited the free HRP while the inhibition by Cs2+ was strong for both free and immobilized HRP. Pb2+ inhibition effect was more on free HRP while Hg2+ and Cu2+ completely inhibited both the free and immobilized HRP. The results indicated that the HRP immobilized on GOMC beads had more resistance towards metal ions than the free HRP.
3.5. Reusability of Immobilized HRP
One of the major advantages of the immobilized enzyme is the reusability, which can effectively reduce the cost of its applications. In this study, the operational stability of the HRP immobilized on GOMC beads was evaluated by performing repeated cycles of ChoX assay. Fig. (7) displays the reusability assay of immobilized HRP for estimation of ChoX. The relative activity of the immobilized HRP reduced with the increasing number of reuses. The immobilized HRP retained ~90% of the residual activity after 12 times repeated reuse in ChoX assay. The enzyme activity obtained in the first cycle was considered as 100% activity. The number of reuses represent a more satisfying performance when compared to recent studies (Table 3). These results may be attributed to the covalent attachment of enzyme to the support after GA activation. Also, the GO coated on the magnetic chitosan beads enhanced the mechanical integrity of the beads, which made them non-brittle and allowed easy separation from the reaction mixture [36]. GO sheet has also been reported as an excellent matrix for enzyme immobilization, which prevented the HRP from easy leach out from the surface of the GOMC beads [37]. The magnetic separation and the enhanced mechanical strength of the chitosan beads proved very practical in the reusability assay.
3.6. Storage Stability
The study of storage stability is a very essential factor to be taken into account for reducing the cost of the immobilized enzyme by the assessment of immobilization efficiency [38]. Free and immobilized HRP were stored in a refrigerator at 4ºC in potassium phosphate buffer (pH = 7) and the enzyme activity was examined at every 10 days interval. The enzyme activity at day zero was considered as 100% and the relative activity was determined. It is evident in Fig. (8), after 30 days of storage; the relative activity of immobilized HRP was 93.72%, whereas that of the free HRP, was ~72%. After 60 days of storage, the relative activity of the immobilized HRP was 60.97%, which was quite better than the free HRP (~50%). The free HRP lost about 72% activity, whereas the immobilized HRP retained more than 70% of its relative activity and showed good stability upon storage for 90 days. Table 4 displays a comparative analysis of the storage stability of HRP immobilized on different carriers, which demonstrated excellent storage stability of HRP immobilized on GOMC beads. These results indicated that the storage stability of the HRP immobilized on GOMC beads greatly enhanced after immobilization, due to the covalent attachment of the enzyme with the carrier support.
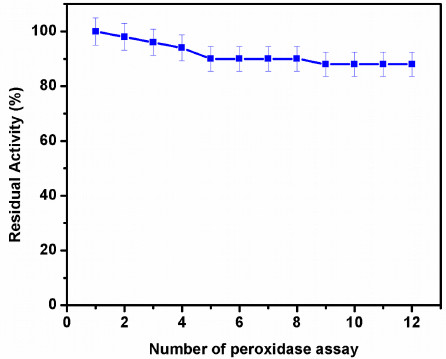
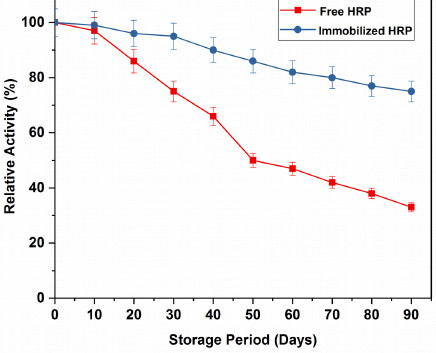
CONCLUSION
In this paper, the preparation of GOMC beads followed by its characterization was studied. Covalent immobilization of HRP onto the surface of GOMC beads was accomplished with GA activation and the application of PGOMC beads for cost-effective ChoX assay was investigated. The immobilization of HRP was successful with ~80% immobilization efficiency and the maximum amount of bound protein was 0.08 mg per bead. The immobilized HRP retained ~90% of the residual activity after being used for up to 12 cycles of ChoX assay, which gave a more satisfying performance as compared to the previous reports, thus providing an economic advantage for the laboratories working on the fermentative production and purification of ChoX. By increasing the reusability of HRP, we took the initiative of using low-cost technology for HRP mediated estimation of ChoX. This approach will be further helpful in reducing the cost of other oxidase based assay methods where HRP is used to catalyse the H2O2 liberated in the reaction. GO has an excellent GOMC which proved to be the most suitable carrier support for carrying out further studies on co-immobilization of ChoX with HRP, which will help provide insights into the development of cholesterol biosensor.
LIST OF ABBREVIATIONS
HRP | = Horseradish Peroxidase |
MNPs | = Magnetic Nanoparticles |
NPs | = Nanoparticles |
GO | = Graphene Oxide |
MC | = Magnetic Chitosan |
GOMC | = Graphene Oxide Coated Magnetic Chitosan |
PGOMC | = Peroxidase immobilized Magnetic Chitosan beads |
GA | = Glutaraldehyde |
XRD | = X-Ray Diffraction |
FTIR | = Fourier Transform Infrared Spectroscopy |
VSM | = Vibrating Sample Magnetometer |
ChoX | = Cholesterol Oxidase |
ETHICS APPROVAL AND CONSENT TO PARTICIPATE
Not applicable
HUMAN AND ANIMAL RIGHTS
No animals/humans were used for studies that are the basis of this research.
CONSENT FOR PUBLICATION
Not applicable
AVAILABILITY OF DATA AND MATERIALS
Not applicable.
FUNDING
None.
CONFLICT OF INTEREST
The authors declare no conflict of interest, financial or otherwise
ACKNOWLEDGEMENTS
Authors acknowledge the School of Biochemical Engineering, Indian Institute of Technology (Banaras Hindu University), Varanasi, India for providing the infrastructural support and research facility for carrying out this research work. We are also thankful to the Central Instrument Facility, IIT BHU for providing the SEM, FTIR and XRD analysis.
The first author is thankful to the Ministry of Human Resource and Development, Govt. of India for providing financial assistance in the form of Teaching Assistantship.