All published articles of this journal are available on ScienceDirect.
Immunofluorescence Assay Using Monoclonal and Polyclonal Antibodies for Detection of Staphylococcal Enterotoxins A in Milk
Abstract
Objectives:
Staphylococcus aureus is a Gram-positive microorganism. S. aureus can grow in various foods and cause food poisoning by secreting enterotoxins. The most common enterotoxins involved in food poisoning are staphylococcal enterotoxin A and staphylococcal enterotoxin B, but Staphylococcal Enterotoxin A (SEA) is predominant. The main types of food contaminated with SEs are meat and meat products, poultry and eggs, milk and dairy products. The aim of this study was to develop a rapid and sensitive fluorescence immunoassay for detection of staphylococcal enterotoxin A in milk.
Methods:
Monoclonal and polyclonal antibodies for SEA were produced and characterized. Competitive fluorescence immunoassay based on Magnetic Nanoparticles (MNPs) was performed and optimized. MNPs were used as a solid carrier of the antibodies. The first step of the assay was immunoreaction between the immobilized antibody onto MNPs and SEA in milk sample. Then the fluorescein-SEA conjugate was added to the sample. Thus, competitive immunoreaction between MNP-mAb/MNP-pAb with SEA and SEA-FITC was performed. These immuno-complexes were separated by a magnetic separator and the obtained supernatants were analyzed. The fluorescent signal from the excess of conjugated SEA was proportional to the SEA contained in the milk. The assay duration was only 30 min.
Results:
The fluorescence immunoassays performed with polyclonal antibody had linear ranges from 5 pg/mL to 100 ng/mL SEA in a buffer, and from 50 pg/mL to 50 ng/mL SEA in spiked milk samples. While the same assays performed with monoclonal antibody had linear ranges from 1 pg/mL to 20 ng/mL SEA in buffer, and from 10 pg/mL to 10 ng/mL SEA in spiked milk samples. The detection limits of the developed immunoassays performed in milk were: 48 pg/mL with polyclonal antibody and 9 pg/mL with monoclonal antibody.
Conclusion:
A rapid and sensitive fluorescence immunoassay based on magnetic nanoparticles with a polyclonal and monoclonal antibody for determination of staphylococcal enterotoxin A in milk was developed.
1. INTRODUCTION
Staphylococcus aureus is a Gram-positive microorganism. It is usually found in nasal passages and skin of healthy individuals. S. aureus can adapt to grow in various foods and cause food poisoning by secreting enterotoxins [1, 2]. Staphylococcal Enterotoxins (SEs) are proteins with 220 240 amino acids and have similar molecular weights (25–30 kDa). The most common SEs involved in food poisoning are SEA and SEB, and SEA is predominant [2, 3]. The main types of food contaminated with SEs are meat and meat products, poultry and eggs, milk and dairy products [2].
There are several types of developed assays for SE determination in food. Each of the methods has its own advantages and disadvantages. A PCR-based molecular-biological analysis is reliable, but it is not a direct method and does not detect SE itself [2, 4]. Chromatographic methods [5] are efficient and sensitive, but the pre-treatment steps, expensive equipment, and trained staff make them unsuitable. A number of rapid and cost-effective immunoassays have been developed for detection of toxins in food products. The most common ones are based on enzyme-linked immunosorbent assay (ELISA) [6, 7], and fluorescent immunoassay (ELFA) [8, 9]. In immunoassays, the antigen-antibody reaction is specific and determines the sensitivity and specificity of the developed method [10, 11]. Furthermore, there are commercially available ELISA kits for SE detection in ng/g levels [5, 6, 12, 13]. They are also lateral flow immunoassays developed for rapid detection of Staphylococcus aureus enterotoxin A, but they give semi-quantitative results [14, 15]. Immunoassay is also suitable for the analysis of milk [16]. Milk samples can be analyzed after only dilution or protein precipitation, without sample preparation. For this reason, immunoassays are important for detection of chemical contaminants in milk.
Magnetic nanoparticles (MNPs) are recently used in immunoassay, in general, ELISA of food-safety fields and medicine [17-19]. MNPs are based on nanoscale materials that have high recognition element densities due to the large relative surface area [20]. Nanotechnology detection systems vary in their analysis schemes but they share the same goal – timely and accurate detection of trace contaminants [21, 22]. Complexes between the nanoparticles and antibodies are formed by covalent immobilization. The immobilized antibody can bind with the antigen in a sample. In recent years, there have been a significant number of publications combining MNPs and ELISA to identify different contaminants [23-26]. There are no publications on combining MNPs and Fluorescence Immunoassay (FIA) for determination of SEA. Integration of MNPs in FIA is a preferred assay, which ensures the advantages of pseudo-homological phase immunological reactions, reduced detection time and improved sensitivity.
In this study, monoclonal and polyclonal antibodies for SEA were produced. The obtained antibodies were characterized and were used for developing a rapid and sensitive MNP-based FIA for determination of SEA in milk.
2. MATERIALS AND METHODS
2.1. Materials
Staphylococcal enterotoxins (SEA, SEB, SEC, SED, SEE), Freund’s adjuvant, Tween 20, Tween 80, bovine serum albumin, polyethylene glycol 4000, ammonium sulfate, dimethylsulphoxide, fetal calf serum, 8-azaguanine, L-glutamine, HiTrap Protein G HP column, anti-sheep IgG whole molecule – peroxydase antibody, anti-mouse IgG whole molecule – peroxidase antibody, 3,3',5,5'-tetramethylbenzidine, 3% H2O2, H2SO4, dimethylformamide, fluorescein 5(6)-isothiocyanate, Sephadex G25 Medium, 2-amino-2-(hydroxymethyl)-1,3-propanediol (Tris), glycine, goat anti-mouse IgG antibody conjugated with alkaline phosphatase, (3-aminopropyl)triethoxysilane (APTES), glutaraldehyde, HiPrepTM 26/10 Desalting, and 1-stepTM NBT/BCIP substrate solution were delivered by Sigma-Aldrich, Germany. Female 8 weeks old Balb/c mice were obtained from The Jackson Laboratory (Bar Harbor, Maine, USA). Dulbecco’s Modified Eagle’s Medium and serum-free CHO medium were taken from Gibco, Gaithersburg, MD. Myeloma cells F0 (ATCC CRL-1646) were purchased from ATCC, Germany. Hypoxanthine-aminopterin-thymidine (HAT) selection medium was taken from USBiological Life Science, US, Canada. Nitrocellulose membrane (0.45µm) was purchased from Scheicher & Schuell, Dassel, Germany.
2.2. Production of Anti-SEA Polyclonal and Monoclonal Antibody
2.2.1. Polyclonal Antibody (pAb)
A single sheep (1 year old, 50 kg) was vaccinated with 7 doses of SEA. Injections were given once every two weeks. First three doses containing increasing SEA concentrations (0.5, 1.0 and 3.0 μg in sterile saline) were injected subcutaneously. The other four doses were antigen-adjuvant emulsion. The SEA solutions (3.0, 5.0, 8.0 and 11.0 μg in sterile saline) emulsified in Incomplete Freund’s adjuvant were injected intramuscularly. After a 2-week rest period, the sheep was made to bleed. Indirect ELISA antiserum titration was performed with negative sheep serum before the first injection, before the third dose and with the final positive sheep antiserum.
2.2.2. Monoclonal Antibody (mAb)
All animal experiments were carried out in accordance with EU Directive 2010/63/EU for animal experiments. Immunization was performed precluding unnecessary pain and distress and to minimize infection.
The group of Balb/c mice (6 animals) was immunized with SEA emulsified with Freund’s adjuvant. SEA (2 μg) was solved in Phosphate-Buffered Saline (PBS), mixed with equal volumes of Complete Freund’s adjuvant, and 0.2 ml was injected intraperitoneally to mice. The prime-boosts were performed every 3 weeks with the same antigen emulsified with Incomplete Freund’s adjuvant. Every week, the animals were bled and the sera tested for antibody development. When a sufficient antibody titer was achieved in serum, immunized mice were sacrificed, the spleens and lymph nodes removed and the lymphocytes were isolated for fusion with myeloma cells.
The myeloma cells (F0; ATCC CRL 1646) were cultivated in Dulbecco’s Modified Eagle’s Medium (DMEM) containing 10% Fetal Calf Serum (FCS) at 37°С and 5% CO2. A week before the fusion, myeloma cells were cultivated in 8-azaguanine.
Selected mice with high immunoglobulin G (IgG) antibody titer against SEA were boosted intraperitoneally 3 days before fusion with 1 µg of antigen dissolved in PBS without adjuvant. Spleens and all lymph nodes from terminal animals were taken and the monocellular suspension was isolated by cell strainers (BD Biosciences, Erenbodegem, Belguim). The cells were incubated in DMEM supplemented with 10% FCS, 4 mM L-glutamine, and antibiotics.
The hybridoma was created by standard laboratory protocol with modifications [27]. Single lymphocytes from the spleens and lymph nodes were fused with the myeloma cells by co-centrifuging in the presence of 50% polyethylene glycol 4000. After the fusion, the cells were cultivated in Hypoxanthine-Aminopterin-Thymidine (HAT) selection medium which allowed only the fused cells to survive in culture. After selection, the final fusion cell culture was resuspended in DMEM supplemented with 20% FCS and the cells were distributed into the 96-well culture plates containing peritoneal macrophages derived from normal mice as feeder cells and incubated at 37°C, 5% CO2. Two weeks later, the culture supernatants of hybridoma growing were screened by ELISA for anti-SEA antibodies secretion. The hybridoma cell clones with high titers were sub-cloned for obtaining stable single-cell derived colonies. Three sub-clones were finally selected and were frozen (5 × 106 cells) in liquid nitrogen in 80% DMEM, 10% FCS, and 10% dimethylsulphoxide. The mouse hybridoma producing anti-SEA antibody was adapted to grow in the serum-free CHO medium. Antibodies from the supernatant were obtained by 50% ammonium sulfate precipitation and subsequent dialysis.
2.3. Titration of Anti-SEA Polyclonal and Monoclonal Antibody in Serums
Indirect ELISA for antiserum titrations (mouse and sheep serums) was performed as described in our previous paper [28]. Briefly, a solution of SEA 5 μg/mL in 50 mM carbonate-bicarbonate buffer, with pH 9.6, was prepared and was equilibrated overnight at 4°C. On the next day, 100 μL of the SEA solution was placed in the wells of a flat-bottomed microtiter plate. After 2 hours adsorption in a shaker, at 37°C, the wells were washed three times with 50 mM PBS pH 7.4 (200 μL). Blocking solution (200 μL in a well), containing 50 mM PBS pH 7.4 and 1% Bovine Serum Albumin (BSA), was added and the plate was incubated 60 imn, at 37°C, in the shaker. The wells were washed as aforementioned. After that, different dilutions (1:1, 1:10, 1:50, 1:250, 1:1 250, 1:6 250, 1:31 250, 1:156 250, 1:781 250, and 1:3 906 250) of antiserums were prepared in 50 mM PBS pH 7.4, containing 1% BSA and 0.05% Tween 20 (PBST-1%-BSA), and 100 μL of them was added in the wells. Subsequent incubation for 60 min, at 37°C, in the shaker was performed. The wells were washed again. Then for samples with primary mAb, secondary anti-mouse IgG antibody–peroxidase was used, diluted to 1:40 000 in PBST-1%-BSA, and for samples with primary pAb, anti-secondary sheep IgG antibody–peroxidase was used, diluted to 1:10 000 in the same buffer (as recommended by the manufacturer). The solutions of secondary antibody (100 μL) were added in the wells and incubated for 60 min, at 37°C, in the shaker. After that, the wells were washed. Then 100 μL of substrate solution (0.5 mL of 1 mg/mL 3,3',5,5'-tetramethylbenzidine in dimethylformamide, 15 μL 3% H2O2, 4.5 mL 50 mM citrate buffer pH 5.0) was added in a well. The plate was incubated in the shaker at 37°C, 15 min, and the reaction was stopped with 2 M H2SO4 (50 μL in a well). The samples were analyzed in a microplate reader Rayto RT-2100C (at 450 nm wavelength).
2.4. Purification of Anti-SEA Polyclonal and Monoclonal Antibodies
The obtained polyclonal and monoclonal antibodies were IgG type. Therefore, an apparatus ÄKTAprime Plus equipped with HiTrap Protein G HP column was used for antibody purification, according to the manufacturerʼs instructions. Finally, buffer exchange was performed with HiPrepTM 26/10 Desalting column. The procedure was described in detail in our previous paper [28].
2.5. Preparation of Fluorescent Conjugates
Three different protein-fluorescent dye conjugates were obtained. Fluorescein 5(6)-isothiocyanate (FITC) was used for a fluorescent marker in all of the conjunctions. The protein in the first conjugate was SEA, in the second one, it was mAb, and in the third – pAb. The protein (0.5 mg) was dissolved in 200 μL of 500 mM carbonate-bicarbonate buffer pH 9.6 and 80 μL of FITC (1 mg/mL in dimethylformamide) was added dropwise. The protein/FITC ratio in the mixtures was 1 mg protein to 0.16 mg FITC. The reaction was performed at 4°C overnight and protected from light. The obtained conjugates were purified by size-exclusion chromatography with a Sephadex G25 Medium column (10 × 155 mm). The flow rate was 0.5 mL/min and fractions were 2 mL each. The elution was performed with 10 mM Tris, containing 150 mM NaCl, pH 8.2. The absorbance of each fraction was measured at 280 nm and 495 nm by a UV/Vis spectrophotometer Jenway 6900. The purity of the obtained protein-FITC fluorescent conjugates was proved by UV/Vis and fluorescent analyses of the conjugates containing fractions. The fluorescent analyses were performed by a Perkin Elmer LS45 fluorescence spectrophotometer. The protein concentration in each of the fluorescent conjugates (SEA-FITC, mAb-FITC, and pAb-FITC) was determined by Bradford assay [29].
2.6. Determination of Activity of Anti-SEA Polyclonal and Monoclonal Antibodies by Direct FIA
Different SEA concentrations (from 0.001 to 1 000 000 ng/mL, in 10-fold dilution) in 50 mM carbonate-bicarbonate buffer pH 9.6 were prepared and equilibrated. Then, 100 μL of the SEA solutions was let to adhere onto wells of a microtiter plate, at 4°C overnight. The excess of toxins was washed with 50 mM Phosphate Buffer Saline (PBS) pH 7.4 (3 times, 250 μL). After that, unoccupied spaces onto the wells were blocked with 250 μL of 50 mM PBS pH 7.4 containing 1% BSA, for 60 min at 37°C in a shaker. The washing step was performed again. Then, the fluorescent conjugate (mAb-FITC or pAb-FITC) was added with concentration 7 μg/mL in 50 mM PBS pH 7.4 containing 0.05% BSA and 0.05% Tween 20.The antigen-antibody reaction was performed for 60 min at 37°C in a shaker. The excesses of the fluorescent conjugates were collected in vials, and their emissions were measured by Perkin Elmer LS45 spectrophotometer, at excitation 495 nm and emission 525 nm, 800 V.
2.7. Sodium Dodecyl Sulfate PAGE and Western Blotting of Purified Anti-SEA Monoclonal Antibody
Western blot assay was performed with a purified monoclonal antibody. Three samples (100 μg/mL SEA) were heated at 96°C for 4 min and loaded onto SDS-PAGE gel electrophoresis. After that, the separated proteins were transferred to nitrocellulose membrane (0.45 µm) by using a Mini Transfer Blot system (BioRad, Richmond, CA) in a buffer containing 48 mM Tris, 110 mM glycine and 20% (v/v) methanol. The membrane was blocked overnight at 4°С in Tris-buffered saline (TBS, pH 7.4) containing 1% skimmed milk, 0.05% Tween 20 and further incubated in different dilutions of the mAb with concentrations 1.00, 0.40 and 0.16 μg/mL. Subsequent incubation with goat anti-mouse IgG antibody conjugated with alkaline phosphatase (5 μg/mL in PBS) was performed and the reaction was visualized with substrate solution, containing nitro-blue tetrazolium and 5-bromo-4-chloro-3'-indolyphosphate (1-stepTM NBT/BCIP substrate solution) [30].
2.8. Determination of Specificity of Anti-SEA Polyclonal and Monoclonal Antibodies
The specificity of the polyclonal and monoclonal antibodies was determined by indirect ELISA. The assay was performed with a purified polyclonal and monoclonal antibody (pAb and mAb). The steps of the analysis were as described above (Titration of Anti-SEA Polyclonal and Monoclonal Antibody Serums). Solutions of five different SEs (SEA, SEB, SEC, SED, SEE) were added to wells of a microtiter plate. The concentrations of the SE solutions were 5 μg/mL, 500 ng/mL and 5 ng/mL. The used concentration of the purified antibodies (pAb and mAb) was 500 ng/mL.
2.9. Development of MNP-Based FIA with Anti-SEA Polyclonal and Monoclonal Antibodies for SEA Determination in Milk
2.9.1. Antibody Immobilization Onto MNPs
MNPs (Fe3O4) were used as a solid carrier of the anti-SEA antibody (mAb and pAb). Nanoparticles were obtained in our laboratory as described in a previous paper [31] with a chemical reactor (from Sirius Company). After that, APTES-modification was performed for the formation of amino groups onto MNP surface [32]. The modified MNPs had an average diameter 8.2 nm, confirmed by transmission electron microscopy (JEM-1400 PLUS). The antibody immobilization was performed by glutaraldehyde activation as described in our previous paper [33]. MNPs (5 mg) were suspended in 5% glutaraldehyde in 50 mM Phosphate Buffer (PB) pH 8.0 (1 mL). The activation was for 120 min, at room temperature, in a shaker. Then, MNPs were washed in 50 mM PB pH 8.0 and 10 mM PB pH 7.4 (× 1 mL), 3 times in each buffer. Antibody (mAb or pAb) solution 1 mg/mL in 10 mM PB pH 7.4 (1 mL) was added to the MNPs. Immobilization was performed at 4°C, overnight. The immobilized MNPs were washed 3 times with 10 mM PB pH 7.4 (1 mL) to remove the excess of the antibody. Thus, MNPs with immobilized monoclonal anti-SEA antibody (MNP-mAb) and MNPs with immobilized polyclonal anti-SEA antibody (MNP-pAb) were obtained. Bradford assay [29] was used for determination of the amount of antibody immobilized onto MNPs. Finally, 1 mL of blocking solution (5% BSA in 10 mM PB pH 7.4 and 0.05% Tween 80) was added and was incubated for 60 min at room temperature, on the shaker. The immobilized nanoparticles were washed another 3 times (× 1 mL 10 mM PB pH 7.4).
2.9.2. Standard Solutions of SEA
Standard solutions of SEA in the buffer and in raw cow milk with 3.5% fat were prepared. All of the standard solutions were analyzed after overnight equilibration at 4°C. The used buffer and milk dilutions for SEA analyses were optimized previously [33].
Optimization of the buffer content was performed for the present mAb and pAb SEA analyses. Different SEA concentrations, ranging from 0.001 to 150 ng/mL, in three buffers were analyzed: 10 mM PB pH 7.4, containing 0.05% Tween 80 (PBT); 10 mM PB pH 7.4, containing 0.05% Tween 80 and 0.05% BSA (PBT-0.05% BSA); 10 mM PB pH 7.4, containing 0.05% Tween 80 and 0.5% BSA (PBT-0.5%BSA). The raw cow milk was diluted with PBT-0.5%BSA at a ratio 1:5. The SEA concentrations in the milk solutions were the same (from 0.001 to 150 ng/mL).
2.9.3. Optimization of the Amount of MNP-pAb and MNP-mAb Relative to the Concentration of the SEA-FITC Conjugate
The amount of antibodies immobilized onto MNPs (MNP-pAb and MNP-mAb) used in the developed assay was optimized. Six different concentrations of the pAb: 0.3, 0.6, 0.9, 2.25, 4.5, 6.75 μg, were loaded in vials. Those amounts were equal to 9.7, 19.3, 29.0, 72.6, 145.2, 217.7 μg MNP-pAb, respectively. Then, five different concentrations of the fluorescent conjugate SEA-FITC: 0.625, 1.25, 2.5, 5.0, 10.0 μg/mL were added to each amount of the antibody (200 μL). After 15-min incubation at 37°C in a shaker, the excess of the conjugate in the supernatant was collected, using a magnetic separator for nanoparticle separation from the reaction mixture.
The same optimization was performed for the monoclonal antibody immobilized onto MNPs (MNP-mAb). The used concentrations of the mAb were: 2, 4, 6 μg, which were equal to: 67, 133, 200 μg MNP-mAb, respectively. Each of those amounts of MNP-mAb was tested with three different concentrations of the fluorescent conjugate SEA-FITC: 2.5, 5.0, 10.0 μg/mL.
The emission of the conjugate excess was measured by a Perkin Elmer LS45 fluorescence spectrophotometer, with excitation 494 nm, emission 518 nm, and voltage 800 V.
2.9.4. SEA Detection by MNP-Based FIA
A competitive FIA was performed for SEA detection in spiked standard buffer and milk solutions. Amounts of immobilized antibody onto MNPs used in the analyses were: 4 μg for mAb and 2.25 μg for pAb. MNP-mAb or MNP-pAb was placed in a series of vials. Standard solutions of SEA (in buffer or in milk) were added (200 μL in a vial). After 15 min incubation in a shaker at 37°C, SEA-FITC conjugate was added to final concentration 2.5 μg/mL, prepared in the same buffer as the SEA samples. Subsequent incubation at the same conditions was performed. Thus, a competitive immunoreaction between MNP-mAb/MNP-pAb with SEA and SEA-FITC was performed. These immuno-complexes were separated by a magnetic separator and the obtained supernatants were collected and analyzed. The supernatants contained the excess of the fluorescent conjugate SEA-FITC in the samples, so fluorescence measurements were performed (Perkin Elmer LS45 spectrophotometer, excitation 494 nm, emission 518 nm, 800 V). The obtained fluorescence intensity was converted in normalized signal (NS%) by following equation: NS = (B0 – B)/(B0 – Bx)×100, where B0 is the fluorescent signal of initial conjugate SEA-FITC solution, B is the fluorescent signal of the sample with SEA, and Bx is the fluorescent signal of the sample without SEA. The NS is inversely proportional to the spiked SEA concentration in the sample.
Recovery analysis of spiked milk samples was also performed. The milk (1:5 dilutions in PBT-0.5% BSA) was spiked with 0.5, 2.0, 10.0 ng/mL of SEA. The samples were treated as described above. The SEA concentration in the spiked sample was calculated based on the standard curve obtained with the standard milk solutions.
3. RESULTS AND DISCUSSION
3.1. Production and Purification of Polyclonal and Monoclonal Antibodies
A polyclonal antibody for SEA was produced in a sheep. The immunogen (SEA) was injected using a series of seven doses. Before each injection, the serum was titrated. The antibody titer represents the last serum dilution in which the antibody is detected. Antibody titer indicates the level of antibodies in blood serum, defined as the greatest dilution (lowest concentration) of the serum at which an antibody assay (ELISA), still produces a detectable positive result. The higher the antibody concentration in the blood, the greater the dilution that will produce a detectable signal. When the positive serum had a sufficient antibody titer, the sheep bled. Antibody titers of the obtained antiserums were observed by indirect ELISA with antigen (SEA) coated microtiter plates. Different dilutions of antiserums were added in the SEA coated wells. Then, the conjugate of secondary antibody with peroxidase was added. The polyclonal anti-SEA antibody titer at 28 days was 1:1000, but after the end of the injection period, the antibody displayed a very high titer up to 1:156 250 (Fig. 1).
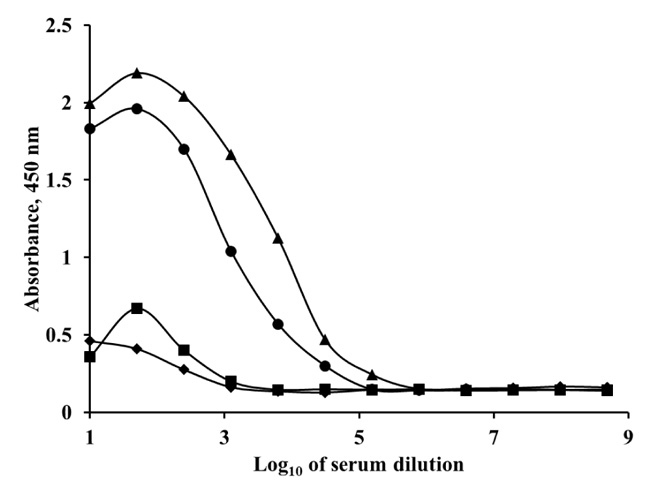
Balb/c mice were immunized with SEA, to obtain monoclonal antibody for the enterotoxin A. Single lymphocytes from the spleens and lymph nodes were fused with the myeloma cells. Hybridoma cell clones with high titers were sub-cloned for stable production of monoclonal antibody.
The monoclonal antibody titer in the obtained antiserum was observed by indirect ELISA (Fig. 1). The antiserum dilutions were the same as in the ELISA experiments with polyclonal antibody. After the end of the injection period, the monoclonal anti-SEA antibody titer increased to 1:31 250. The obtained pAb and mAb were IgG type. The purification of both the antibodies was performed by affinity chromatography with Protein G Sepharose High-Performance resin. The IgG type antibodies were eluted in the second peak of the chromatograms.
3.2. Determination of Activity and Specificity of Polyclonal and Monoclonal Antibody by Direct FIA
The activity of the obtained anti-SEA polyclonal and anti-SEA monoclonal antibody to SEA was determined by direct FIA (Fig. 2). First, fluorescent conjugates of polyclonal and monoclonal antibody were prepared for direct FIA development. The amino groups of the antibody are able to couple to isothiocyanate groups of FITC, resulting in amide bonds. The obtained fluorescent conjugates were proved by UV-Vis and fluorescence spectrophotometric analysis (data not shown). The ratio of molar fluorescent dye to antibody in the conjugate Ab-FITC is essential for further analyses performing and comparison. The concentration of antibody in the obtained conjugate was determined by Bradford assay [29]. Degree of labeling of the antibodies was determined by molar Fluorescent dye per Protein ratio (F/P). Both the fluorescent conjugates (mAb-FITC and pAb-FITC) had F/P 3.5. That F/P ratio provided optimal signal-to-noise ratio and reproducible results for the assay [34].
Fig. (2). shows that the linear range of direct FIA performed with pAb-FITC fluorescent conjugate is from 0.1 ng/mL to 10 μg/mL. The linear equation is y = -6.732ln(x) + 18.286 and correlation coefficient R2 = 0.9870. The coefficient of variation (CV) of that assay ranges from 2.2% to 9.5%. The linear range of direct FIA performed with mAb-FITC fluorescent conjugate is from 0.01 ng/mL to 10 μg/mL. The equation is y = -7.321ln(x) + 29.381, R2 = 0.9892. The CV of that assay ranges from 2% to 10%. The obtained results show that the two obtained antibodies have high activity and sensitivity.
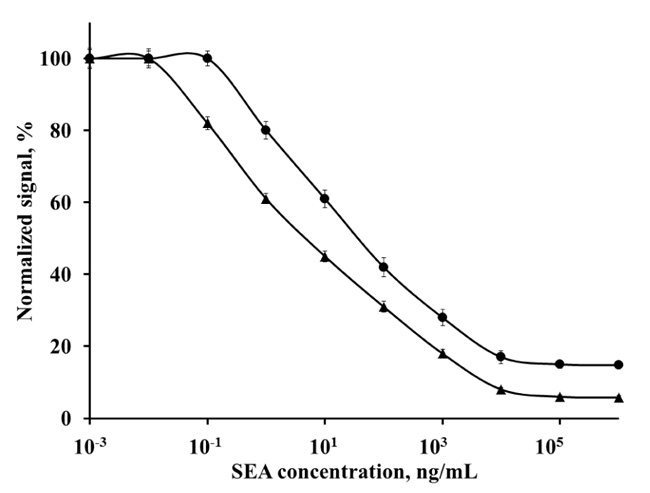
The activity of the purified monoclonal antibody against SEA was analyzed also by Western blot. The results showed dose-dependent binding of the antibody to the target molecule. The monoclonal antibody had reactivity against SEA even at low antibody concentration (0.16 μg/mL), which confirmed the quality of the obtained antibody.
The specificity of the obtained antibodies was evaluated. The various SEs share a certain degree of homology. Therefore, solutions of SEB, SEC, SED and SEE were analyzed with the obtained antibodies (mAb and pAb) by indirect ELISA. No cross-reactivity was observed with both antibodies with standard concentrations below 500 ng/mL SEs. A 0.05% cross-reactivity was observed with SEE at the highest test concentration 5 μg/mL, analyzed with the pAb. That was probably due to 81% sequence homology of SEA and SEE [1, 3, 35]. The mAb had no cross-reactivity even at the highest concentration of SEE (5 μg/mL).
3.3. MNPs based FIA with Immobilized Sheep Anti-SEA polyclonal and Mice Anti-SEA Monoclonal Antibodies for SEA Determination in Buffer and Milk
A fluorescent conjugate of SEA was prepared for competitive FIA development with a polyclonal and monoclonal antibody. The enterotoxin is a protein and its amino groups are able to couple to isothiocyanate groups of FITC, resulting in amide bonds. The degree of labeling in the obtained conjugate (F/P) is essential for fluorescent conjugate properties. It was observed that higher F/P (more than 4.0) and lower F/P (less than 1.0) provided lower sensitivity and reproducibility of the assay [34]. It was found that the ratio F/P of SEA-FITC was 3.6 and it was able to provide high assay sensitivity. The obtained SEA-FITC conjugate was used for the competitive MNP-based FIA. The assay also used immobilized antibody onto MNPs. The immunoreaction between the antibody to MNPs and SEA (conjugated or unconjugated) is essential for the analyses. Antibody immobilization procedure was performed by glutaraldehyde, that was used as a cross-linker. The amino groups of antibody were bound with the amino groups of APTES coated MNPs by glutaraldehyde. The coupled amount of antibody onto MNPs after the reaction was determined by Bradford assay [29]. It was represented as μg antibody per 1 mg MNPs. For MNP-pAb, the amount was 31 μg antibody per 1 mg MNPs. Competitive MNP-based FIA was also performed with the mAb. The mAb was immobilized onto APTES-modified MNPs, as described above, by glutaraldehyde linkage. The amount of monoclonal antibody immobilized onto the MNPs, by Bradford assay, was 30 μg antibody per 1 mg MNPs. While performing the assay, there was a competition observed between fluorescent-labeled SEA and unlabeled SEA to bond with the antibody onto the MNPs.
It is necessary to determine in advance the optimal conditions for carrying out the assay by varying the concentrations of the main components of the analysis: the amount of immobilized antibody on magnetic nanoparticles (MNP-Ab); concentration of fluorescence conjugate and the concentration of albumin in the reaction buffer.
For this purpose, samples with different amounts of MNP-pAb, containing 0.3 - 6.75 μg pAb and different concentration of SEA-FITC conjugate (0.625 - 10 μg/mL) were prepared. The change in conjugate emission was calculated before and after the antibody-conjugate reaction (Fig. 3). The results show that the best resolution is obtained at 2.5 μg/mL conjugate and 2.25 μg pAb (equal to 72.6 μg MNP-pAb).
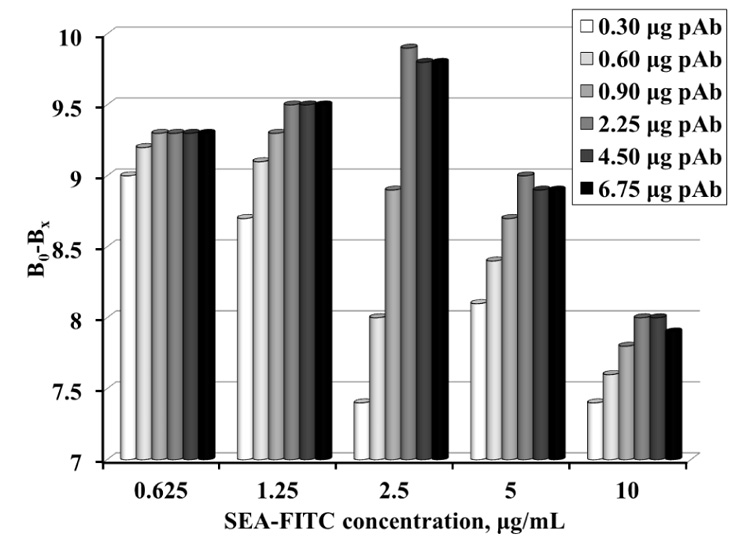
The amount of immobilized mAb according to the concentration of the SEA-FITC conjugate was optimized for MNP-based FIA (Fig. 4). The following amounts of immobilized mAb on MNPs were used: 2, 4 and 6 μg mAb respectively). SEA-FITC concentrations also varied (2.5, 5 and 10 μg/mL).
Incubation of MNP-mAb with the various concentrations of the conjugate in a buffer without SEA was performed and the fluorescence signal was measured. The change in the conjugate emission was calculated before and after the reaction. Fig. (4). shows that the highest resolution of fluorescence signal is obtained at 2.5 μg/mL SEA-FITC conjugate and at 4 μg mAb (equal to 133 μg MNP-mAb).
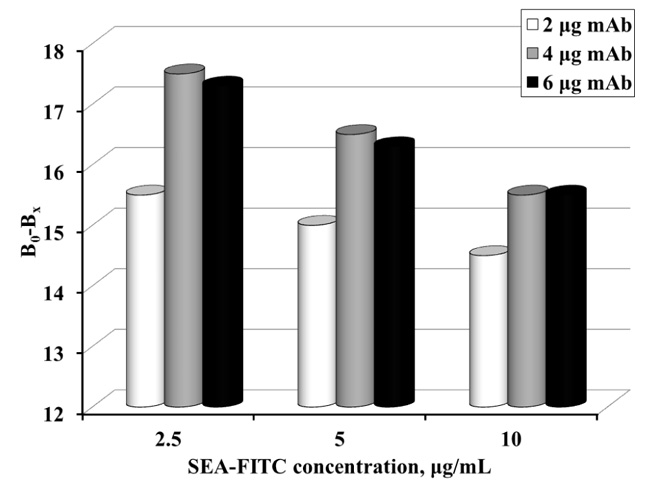
The influence of protein content in the buffer used was tested. Higher albumin concentrations had a positive effect on SEA, probable due to better solubility and stability in these buffers. The comparison of SEA in three buffers with different BSA concentrations and standard milk solutions was made using the optimal amount of MNP-pAb (Fig. 5). The largest linearity and the biggest slop were obtained with PBT-0.5% BSA – from 0.005 to 100 ng/mL SEA. It was found that the optimal buffer was 10 mM PB pH 7.4 containing 0.05% Tween 80 and 0.5% BSA (PBT-0.5% BSA). The linear range of the SEA in milk standard solutions (milk diluted in PBT-0.5% BSA, 1:5) was shorter from 0.05 to 50 ng/mL.
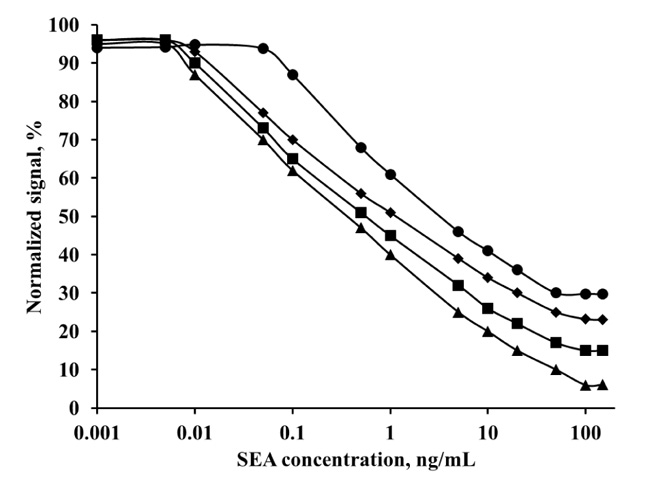
Fig. (6). shows the comparison of the obtained linear ranges with monoclonal anti-SEA antibody in two different concentrations of mAb – 4 μg and 6 μg. At the lower antibody concentration (4 μg), the linear range was from 0.001 to 20 ng/mL SEA (LOD 0.9 pg/mL) in standard buffer solutions.
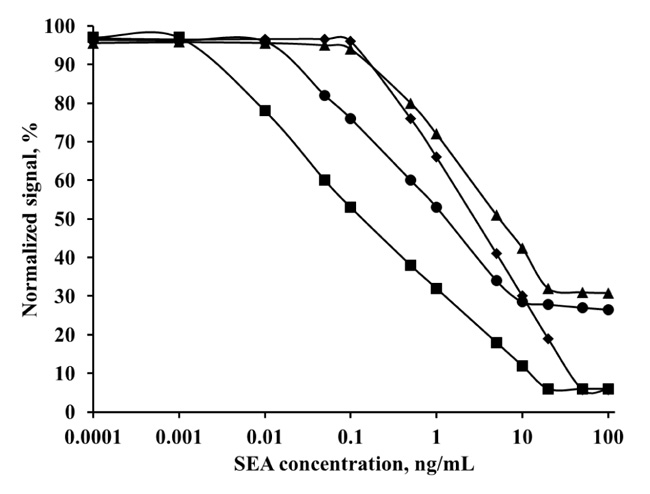
Additionally, results in milk standard solutions had linearity from 0.01 to 10 ng/mL SEA (LOD 9 pg/mL). The linear range with milk samples was shorter than buffer samples, but the slop of the curve was greater, so the assay had better sensitivity. The same analysis, performed with a higher antibody concentration (6 μg), had shorter linear ranges and the LOD shifted to higher SEA concentrations. In that case, the linearity of SEA in buffer standard solutions was from 0.1 to 50 ng/mL, and in milk standard solutions, it was from 0.1 to 20 ng/mL. Consequently, optimal concentration of monoclonal anti-SEA antibody in the assay was 4 μg in buffer SEA solutions and in milk SEA solutions.
3.4. Validation of MNP-Based FIA with Anti-SEA-pAb and Anti-SEA-mAb
In this study, MNP-based FIAs were developed and validated. The assays performed with polyclonal antibody had linear ranges from 5 pg/mL to 100 ng/mL SEA in buffer, and from 50 pg/mL to 50 ng/mL SEA in spiked milk samples. While the same assays performed with monoclonal antibody had linear ranges from 1 pg/mL to 20 ng/mL SEA in buffer, and from 10 pg/mL to 10 ng/mL SEA in spiked milk samples. The polyclonal antibody provided larger linearity of the results but higher LOD (LOD 4.8 pg/mL SEA in buffer and 48 pg/mL SEA in spiked milk samples). Whereas, monoclonal antibody provided shorter linearity of the results, but lower LOD (LOD 0.9 pg/mL SEA in buffer and 9 pg/mL SEA in spiked milk samples). These results are satisfactory and comparable with those obtained by other authors. For example, Upadhyay and Nara [14] described a lateral flow assay that had LOD 0.5 μg/mL SEA in both buffer and spiked milk samples. Lower LOD (1 ng/mL SEA in buffer) had been reported from Haddada et al. [36] obtained by gold colloid-nanostructured surfaces and piezoelectric immunosensing. Also our results were similar to that obtained with a commercial kit (RIDASCREEN®) that had LOD 1 ng/g SEA in food, reported from McCallum and Phillips [37]. Singh et al. [38] developed a dot-blot strip assay with LOD 48 ng/mL SEA. They noticed that the monoclonal anti-SEA antibody had a better ability to capture than polyclonal anti-SEA antibody, but polyclonal antibody had advantages over monoclonal antibody for detection of SEA. They explained this with the multiple paratopes of the polyclonal antibodies and stronger binding to many epitopes on SEA. This observation could be referred to the presented result in our present study, where a larger linear range (5 pg/mL to 100 ng/mL SEA in buffer) was obtained with polyclonal antibody compared to the same assay performed with monoclonal antibody (1 pg/mL to 20 ng/mL). Also in our previous paper [33], the LOD for similar assay performed by a monoclonal anti-SEA antibody (from Sigma Aldrich Company) immobilized onto MNPs was 230 pg/mL SEA in the spiked milk sample. Compared to the results of the present paper (LOD 48 pg/mL SEA in milk detected with polyclonal antibody, and LOD 9 pg/mL SEA in milk detected with monoclonal antibody), the previous assay had a higher LOD, although using monoclonal antibody, probably due to the fluorescent conjugate used for signal detection (previous paper – SEA-ATTO620, present paper – SEA-FITC). Consequently, the present MNP-based FIA is more suitable for SEA detection in milk samples.
The validation of the present assay included determination of intra- and inter-assay precision by calculation of the Coefficient of Variation (CV) (Table 1).
Antibody | Coefficient of Variation, % (Intra Precision), n = 10 | Coefficient of Variation, % (Inter Precision), n = 10 | ||
---|---|---|---|---|
Buffer | Milk | Buffer | Milk | |
Mice anti-SEA mAb | 7.1 | 8.8 | 10.0 | 11.5 |
Sheet anti-SEA pAb | 6.2 | 8.1 | 8.1 | 9.8 |
The intra-assay precision was determined by analyzing each concentration ten times per run at one time. The inter-assay precision was determined by analyzing each concentration ten different times. The coefficients of variation are slightly higher than those obtained in the buffer, and this is fully understandable due to the complex composition of milk. However, the CV values are low and these results indicate that the developed MNP-based method gave accurate and repeatable results [35].
The recovery of three spiked samples with SEA was calculated (Table 2). The recovery of milk samples was obtained in the range of 96% to 120%. These values in SEA milk sample are higher than the ones for SEA buffer sample, due to the different components in milk.
SЕА in Spiked sample, ng/ml |
Detected SEA Concentration, ng/mL |
Recovery, % | ||
---|---|---|---|---|
Buffer | Milk | Buffer | Milk | |
0.5 | 0.49 | 0.48 | 98 | 96 |
2 | 2.02 | 2.1 | 101 | 105 |
10 | 11.0 | 12.0 | 110 | 120 |
CONCLUSION
A rapid and sensitive MNP-based FIA with a polyclonal and monoclonal antibody for determination of SEA in milk was developed. The use of MNPs in immunoassay ensures very low LOD of SEA in milk – 48 pg/mL (for FIA with pAb) and 9 pg/mL (for FIA with mAb). The use of magnetic nanoparticles leads to a reduction in assay time compared to ELISA. The stated advantages of magnetic nanoparticles provide a simple procedure for separating magnetic nanoparticles with the immobilized antibody and bound antigen from the reaction mixture.
ETHICS APPROVAL AND CONSENT TO PARTICIPATE
All animal experiments were performed in accordance with EU Directive 2010/63/EU Bulgaria.
HUMAN AND ANIMAL RIGHTS
No humans were used in this study. All animal experiments in the European Union (EU) are regulated under Directive 2010/63/EU Bulgaria, for the protection of animals used for scientific purposes.
CONSENT FOR PUBLICATION
Not applicable.
AVAILABILITY OF DATA AND MATERIAL
Not applicable.
FUNDING
This study was funded by the National Scientific Fund Research Bulgaria (Project 17/3-2017) .
CONFLICT OF INTEREST
The authors declare no conflict of interest, financial or otherwise.
ACKNOWLEDGEMENTS
Declared none.