All published articles of this journal are available on ScienceDirect.
Bioremediation of Polycyclic Aromatic Hydrocarbon (PAHs): A Perspective
Abstract
Hydrocarbon pollution is a perennial problem not only in India but throughout the globe. A plethora of microorganisms have been reported to be efficient degraders of these recalcitrant pollutants. One of the major concerns of environmental problem is the presence of hydrocarbons due to the various anthropogenic activities. PAHs are ubiquitous in nature i.e. present in soil, water and air. Presence of PAHs in environment creates problem as their presence have deleterious effect on human and animals. They also have the ability to cause the tumors in human and animals. Some of the microorganisms are capable of transforming and degrading these PAHs and remove them from the environment. The present review describes about the sources, structure, fate and toxicity of PAHs as well as different bioremediation techniques involved in the removing of contaminants from the environment which are efficient and cost-effective. The conventional approaches used for removal of PAH are not only environment friendly but also are able to reduce the risk to human and ecosystem.
INTRODUCTION
Polycyclic Aromatic Hydrocarbons (PAHs) are potent environment pollutants which are ubiquitously distributed, having fused aromatic rings [1, 2]. PAHs are formed due to incomplete combustion of wood, coal, oil and gasoline. Presence of PAHs has been reported in crude oil, coal tar and asphalt [3]. Main cause of soil and water pollution is the release of hydrocarbons accidentally or through human activities [4, 5]. PAHs are toxic, carcinogenic and mutagenic so their presence in environment is of great concern and has deleterious effect on human health. 16 PAHs have been listed as priority pollutants by US EPA and are monitored continuously in industrial effluents [6]. Environmental concern has increased and potential techniques have been developed and adopted for overcoming this problem. Bioremediation is a technique which uses microbes (bacteria, fungi and alage) to degrade or transform and mineralize various contaminants to carbon dioxide, water, inorganic salts and other by products. Biodegradation of polycyclic aromatic hydrocarbon (PAHs) has been achieved by bacteria [7, 8] fungi [9, 10] or algae [11, 12].
SOURCES OF PAHs
Large quantities of hazardous chemicals have been generated over the last century due to the development in industrial, agricultural and medical activities leading to the increase in environmental pollution (Fig. 1). Ubiquitous distribution of PAH and their detrimental effect on human health has prompted the interest of various resources in the mechanism of degradation and their possible fate in nature [13, 14]. PAHs (also, known as Polyarenes, Polynuclear aromatic Hydrocarbons) are product of incomplete combustion of organic compounds. PAHs are organic pollutants that are widely distributed in the environment which are toxic and persistent [15, 16]. The major source of energy for industry and daily life in the era of industrialization is obtained from petroleum based products. Hence the chances of spillage and leakage occur regularly during the exploration, production, refining, transport and storage of petroleum products. Generally, PAHs are non-polar, neutral, organic molecules which are ubiquitous environmental pollutants which can be found naturally or anthropogenically [17, 18]. Natural sources include volcanoes and forest fires. Anthropogenic sources include [19].
- Combustion of fossil fuel- including motor vehicle emission and power generation.
- Wood burning.
- Municipal and industrial waste inceration.
- Coal tar, coke, asphalt, crude oil, creosote, asphalt roads, roofing tar.
- Discharges from industrial plants and waste water treatment plants.
- Hazardous wastes sites, coal gasification sites, smoke houses, aluminium production plants.
- Atmospheric contamination of leafy plants.
- Cigarette smoke.
- Charbroiled meat
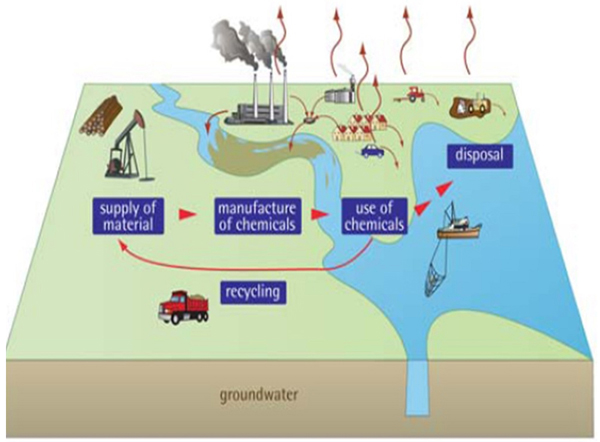
STRUCTURE OF PAHs
PAHs, structure consist of carbon & hydrogen atoms having two or more fused benzene rings in linear, angular or cluster arrangements [20]. The molecular arrangements of PAHs are shown in Fig. (2) [21-23]. PAHs with an angular arrangement are more stable than with linear arrangement [24]. PAHs not only contain carbon & hydrogen but in broader way heterocyclic (heteroatom) i.e. PAH containing N, S and O atoms are also considered to be known as PAH. Generally, molecular weight ranges from 166 to 328. Molecular weight of the compound generally depends on its number as well as position of fused rings and other compounds. PAHs are formed due to thermolysis of various organic molecules in the environment. PAHs are produced as a result of incomplete combustion of organic compound at high temperature (500-800°C) to low temperature (100-300°C) for long duration [25]. The PAH molecule exhibits biochemical persistence because of the presence of dense π electrons on both sides of ring structure. Hence, PAHs compounds are more resistant to nucleophilic attack [26].
PAHs are flat, crystalline solid with high melting and boiling point but with low vapour pressure and water solubility. PAHs range in appearance from colorless to white or pale yellow-green. On the basis of molecular weight the PAHs are classified as Low Molecular Weight (LMW) having two or three fused benzene rings like Naphthalene, Fluroene, and Phenanthrene and High Molecular Weight (HMW) PAHs are those having four or more fused benzene rings like Pyrene [19]. Increase in molecular mass, increases the hydrophobicity/ lipophilicity and decreases water solubility and vapour pressure making the compound become more recalcitrant [27]. So as compared to LMW, HMW are not only poorly bioavailable and recalcitrant to microbial degradation, but are also more carcinogenic, mutagenic and teratogenic [28-30]. On the basis of the genotoxicity and abundance US Environmental Protection Agency (US-EPA) have listed 16 PAH compounds as priority pollutants as shown in Table 1.
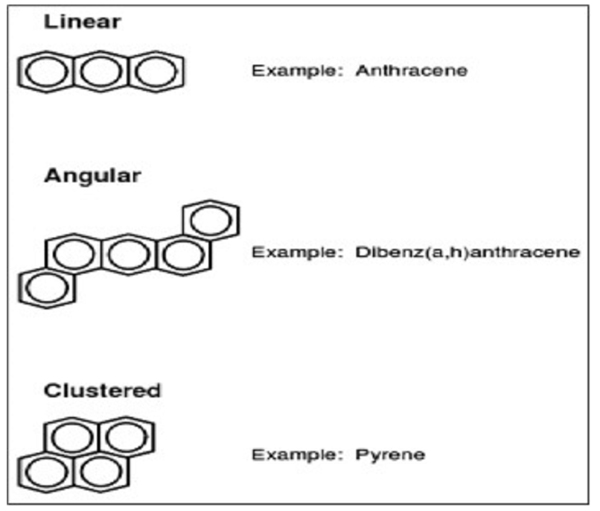
PAH names | Number of rings |
Molecular weight (g mole-1) |
Solubility in water (mg L-1) | Vapor pressure (Pa) | Log Kow |
---|---|---|---|---|---|
Naphthalene | 2 | 128.17 | 31 | 11.866 | 3.37 |
Acenaphthene | 3 | 154.21 | 3.8 | 0.500 | 3.92 |
Acenaphthylene | 3 | 152.2 | 16.1 | 3.866 | 4.00 |
Anthracene | 3 | 178.23 | 0.045 | 3.40 x 10-3 | 4.54 |
Phenanthrene | 3 | 178.23 | 1.1 | 9.07 x 10-2 | 4.57 |
Fluorene | 3 | 166.22 | 1.9 | 0.432 | 4.18 |
Fluoranthene | 4 | 202.26 | 0.26 | 1.08 x 10-3 | 5.22 |
Benz[a]anthracene* | 4 | 228.29 | 0.011 | 2.05 x 10-5 | 5.91 |
Chrysene* | 4 | 228.29 | 0.0015 | 1.04 x 10-6 | 5.91 |
Pyrene | 4 | 202.26 | 0.132 | 5.67 x 10-4 | 5.18 |
Benzo[a]pyrene* | 5 | 252.32 | 0.0038 | 6.52 x 10-7 | 5.91 |
Benzo[b]fluoranthene* | 5 | 252.32 | 0.0015 | 1.07 x 10-5 | 5.80 |
Benzo[k]fluoranthene* | 5 | 252.32 | 0.0008 | 1.28 x 10-8 | 6.00 |
Dibenz[a,h]anthracene* | 6 | 278.35 | 0.0005 | 2.80 x 10-9 | 6.75 |
Benzo[g,h,i]perylene* | 6 | 276.34 | 0.00026 | 1.33 x 10-8 | 6.50 |
Indeno[1,2,3-cd]pyrene* | 6 | 276.34 | 0.062 | 1.87 x 10-8 | 6.50 |
PAHs are also classified on the basis of their ring structure (a) Alternant (b) Non-Alternant. Alternant PAHs include Anthracene, Phenanthrene and Chrysene which are derived from benzene by fusion of additional 6- membered benzoid ring and contain fewer ring than benzoid ring [33]. Non-Alternant PAH includes Fluoranthene which contain rings with fewer than 6 carbon atoms in addition to 6- membered ring. As shown below in Fig. (3) there is difference in two structures. Chrysene consists of four fused, six carbon benzene rings. Fluoranthene, on the other hand, contains naphthalene and a benzene unit connected by a five-membered ring (in the centre of the structure) and is indicative of lower temperature and less efficient combustion.
Most PAHs consist of a “bay-region”, a “K-region” and “L -region”. For example an open inner corner of Phenanthrene structure is Bay-region i.e. internal open inner corner. The K- region is an external closed corner and L-region represents the pair of opposed anthracenic point atoms [34]. These bay- and K-region epoxides are chemically reactive. Thus, they are developed metabolically and biologically. The bay region between carbon atom 4 and 5 is sterically hindered in Phenanthrene. The most oleinic aromatic double bond with high electron density is the K region where there is a presence of double bond between carbon 9 and 10 (Fig. 4)[35].
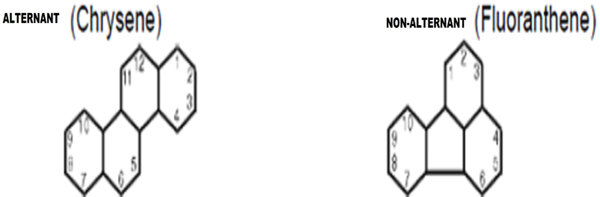
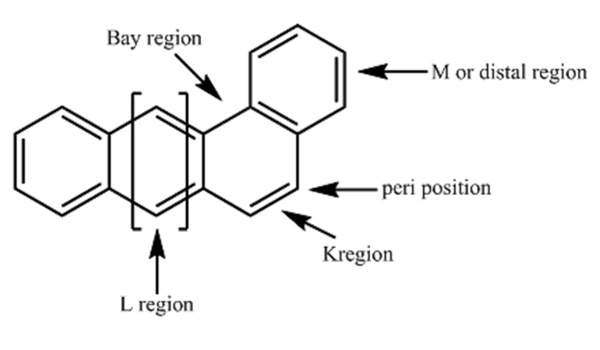
SOLUBILITY OF PAHs
As discussed earlier solubility of any compound in water is dependent on temperature, pH, ionic strength (concentration of soluble salts) other organic chemicals (i.e. dissolved organic carbon) [36]. Solubility can be estimated by 2 ways (a) Chemical Structure (b) Octanol-Water Partition Coefficients.
Chemical Structure: Wilson and Jones [17] reported that (a) with increase in benzene rings in PAH there is a decrease in solubility. Planar PAHs are less toxic and less reactive [37].
(b) Octanol-Water Partition Coefficients (Kow): Octanol-Water Partition Coefficients is expressed as an inverse relationship between Kow and solubility which is determined with the following equation
Kow = Amount of organic chemical in octanol (mg/L)
Amount of organic chemical in water (mg/L).
It is expressed in terms of log Kow. For example: log Kow of a naphthalene is 3.37 where as that of Indeno [1, 2, 3-Cd] Pyrene is 6.50 with lower Kow naphthalene is said to be more soluble. Removal of PAHs from the environment takes place by the process of volatilization, photo-oxidation, chemical oxidation, bioaccumulation, adsorption, microbial degradation and their adhesion to the soil matrix [29, 38-40]. PAHs found in soil can under go number of processes it may get bio accumulated by some organisms, low molecular weight PAHs have a tendency to evaporate and can also get adsorbed onto soil particles and organic matter fraction, which may be lost either by leaching/ degradation [41]. Reid et al. [42], and Semple et al. [41], reported that fate of PAHs in soil is affected by various factors like weather and climate, biological diversity and abundance, amount and nature of the soil/minerals which include properties like molecular structure, polarity, solubility, hydrophobicity, lipophilicity, temperature, pH and moisture content. The persistence of PAHs is also influenced with the ‘age’ of the co-existing contaminants in the soil matrix. If the age of coexisting contaminants is higher the persistence of PAHs is longer. However, PAHs in the environment can be removed through a natural techniques using microbial degradation or using physical or chemical processes as shown in Fig. (5)[43]. Phenanthrene has shown reduced biodegradability due to depletion of oxygen and increase of anaerobicity in the environment. However, it has been reported that efficiency of PAH degradation under anaerobic condition is limited [44, 45]. BTEX (Benzene, Toluene, Ethyl benzene and Xylene) are the compounds which are degraded in-situ by microbes, thereby leading to the depletion of oxygen in the surrounding environment and creating an anaerobic condition [44]. The microbial growth is also inhibited in the presence of heavy metals thus limiting the metabolism of the pollutants under anaerobic condition [46].
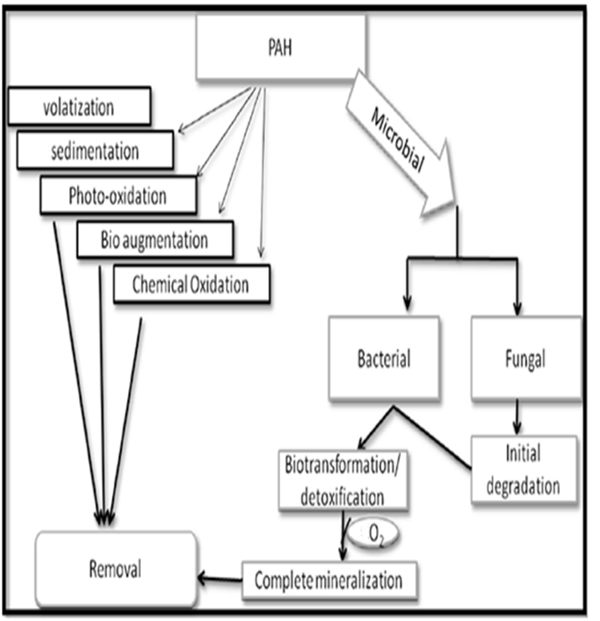
PAH bioavailability for a bioremediation process to be successful the microorganisms, or their enzymes, need to be in physical contact with the organic contaminant. As both the properties of the soil and the type of the contaminant determine bioavailability and bio accessibility [47]. Bioavailability represents the fraction that is taken up by the cell, and causes their toxic effects. Whereas the term bio accessibility means the fraction that is potentially available for biota in soils also known as environmental availability [48]. Two steps are involved in the biodegradation of PAHs in soil. They are (a) uptake of the PAH by microbes (b) break down (metabolism) of the PAH [49]. PAHs degradation, its transport and sequestration is dependent on their bioavailability in environment [50]. The factors responsible for lower degradation of the PAHs are the poor bioavailability which is caused by their lower water solubility, low dissolution rate and strong adsorption to the soil matrix [51]. PAH bioavailability in the environment is affected by various parameters like physical properties, soil characteristics and receptor organisms [12, 52]. Alexander [53] reported that LMW PAHs have the property of volatility, higher solubility and can also be used as a sole source of carbon by micro organisms which could be removed at a faster rate by biological as well as physic-chemical process. Bioavailability of PAHs in the environment changes with time and weathering stage [54]. PAHs bioavailability is influenced by various soil parameters like organic matter content, texture, aggregation and ageing.
TOXICITY OF PAHs
According to US-EPA among all PAHs known Benzo (a) Pyrene is recognized as the most dangerous pollutant as it is extremely carcinogenic and the major component of smoke released from cigarettes [55]. PAHs are quickly absorbed into the gastro-intestinal tracts of humans as they are highly lipid soluble [15, 56, 57]. The toxicity of any PAHs can be measured using LD50 values. It is expressed as milligrams of toxic material per kilograms of the subject’s body weight that will cause death in 50% of cases. Many PAHs lead to toxic effect in living organisms. Due to their carcinogenic and mutagenic nature PAHs are of significant concern as environmental contaminant [58]. It has been reported by Sims and Overcash [58] that LMW i.e. one, two or three-ring compounds are acutely toxic while HMW PAHs are considered as genotoxic [59, 60]. The toxicity of PAHs is induced when there is a transformation to reactive products by chemical or biochemical processes, particularly there is incorporation of oxygen into the PAH ring structure. Photoxidation of PAHs increases the toxicity of the compounds. 16 PAHs have been identified as major pollutants regarding to its potential exposure and adverse health effects on humans and animals [21]. Kannaly and Haryama [61] and Cerniglia [29] reported that the genotoxicity of PAHs increases with increase in number of fused benzene rings. PAHs also have toxic effect on humans which can lead to nephrotoxicity and haemolytic anemia. About 75% of PAHs are absorbed through skin. PAHs has fast absorption rate due to its high potential for biomagnifications in the food chain. The effect of PAHs has also been studied on mammalian health. PAHs are known to have acute carcinogenic, mutagenic and teratogenic properties. Among all PAHs, Phenanthrene is a photo-sensitizer of human skin a mild allergen, and mutagenic to bacterial system. Samanta et al. [14], reported that Phenanthrene is smallest PAH which has Bay and K- region in its structure and hence it is considered as model substrate for studying the metabolism of carcinogenic PAHs. Food may also contain PAHs in varying quantities when exposed to high temperature. It has been suggested in studies that PAHs are also found in oil, fats and cereals. PAHs are also even obtained from cooked meat and vegetables [62]. PAH- contaminated soil is of great concern to human health as it passes into the food chain [63]. The effect on human health will mainly depend on the time and route of exposure as well as on the concentration of the PAHs. Various factor affects human health which include symptoms of eye irritation, nausea, vomiting and diarrhea. PAHs such as Anthracene, Benzo(a) Pyrene and Naphthalene have the ability to cause skin irritation while Anthracene and Benzo(a) Pyrene cause allergic response in animals and humans [29].
PAHs are suspected carcinogens. They become genotoxic when activated by mammalian enzymes to reactive epoxides and quinones by enzymes such as cyctochrome P450 monoxygenase [64]. Harvey [65] reported that the formed intermediates may undergo oxidation and/or hydrolysis before combining DNA for the formation of covalent adducts. The formation of adducts thus leads to mutation resulting in tumors. Movement and fate of PAHs, in the environment is as shown in Table 2.
PAHs DEGRADATION TECHNIQUES
Chemical Degradation
Detecting the persistence of PAH after its degradation in environment is quite difficult. The existence of PAH in anaerobic condition depends on substrate interaction, pH and redox condition [66]. Degradation of PAH in soil occurs through biotic process. Upon undergoing chemical reactions, PAHs are transformed into other derivatives of PAHs. As considerable amount of energy is required to change an aromatic compound into a non- aromatic compound, PAHs do not lose their aromaticity character. The efficiency of PAHs chemical degradation is limited because of their low aqueous solubility and vapour pressure [67]. It has been reported that surfactants have the property to overcome the problems related to PAHs low aqueous solubility [68]. Solubility of PAHs is enhanced in the presence of surfactants by decreasing the interfacial surface tension [69].
Process | Consequence | Factors |
---|---|---|
Transfer (processes that relocate PAHs without altering their structure) | ||
Volatilization | Loss of PAHs due to evaporation from soil, plant, or aquatic ecosystems | Vapor pressure, wind speed, temperature |
Absorption | Uptake of PAHs by plant roots or animal ingestion. Polycyclic aromatic hydrocarbons usually do no transfer into aboveground biomass from soil. | Cell membrane transport, contact time, susceptibility, plant species |
Leaching | Translocation of PAH either laterally or downward through soils | Water content, macropores, soil texture, clay and organic matter content, rainfall intensity, irrigation |
Erosion | Movement of PAH by water or wind action | Rainfall, wind speed, size of clay and organic matter particles with adsorbed PAH on them |
Degradation (processes that alter the PAH structure ) | ||
Biological | Degradation of PAHs by microorganisms, biodegradation and cometabolism | Enviromental factors (pH, moisture, temperature, oxygen), nutrient status organic matter content, PAH bioavailabilty, microbial community present, molecular weight of PAH (LMW or HMW) |
Chemical | Alteration of PAHs by chemical processes such as photochemical (i.e. UV light) and oxidation-reduction reactions | High and low pH, structure of PAH, intensity and duration of sunlight, exposure to sunlight, and same factors as for microbial degradation |
Sequestration (processes that relocate PAHs into long-term storage without altering structure) | ||
Adsorption | Removal of PAHs from bioavailable pools through intraction with soils and sediments | Clay and organic matter content, clay type, moisture |
Diffusion | Diffusion of PAH into soil micropores where it is unavailable for microbial degradation | Hydrophobic nature of micropores and PAH |
BIOREMEDIATION
Bioremediation involves the process of biodegradation and biotransformation where by organic contaminants are transformed or degraded to an environmentally safe levels in ground water and soil [70].The micro-organisms used for the biodegradation may be indigenous to a contaminated area or may be extraneous and brought to the contaminated site. The complete mineralization of the pollutants leads to the generation of CO2, H2O and biomass [71-73].
Phytodegradation
Phytoremediation is an emerging technology that uses various plants to degrade, extract, contain or immobilize contaminants. There are various mechanism like volatilization, rhizoremediation, phytotransformation, phytostabilization, hydraulic control that are used for contaminant of pollutants from air, soil and water. Phytodegradation is also called as Phyto transformation which involves degradation of organic contaminants to simple molecules or the incorporation of these molecules into plant tissues [74, 75]. Adam & Duncan [76] reported the use of various grasses and leguminous plants having ability to phytodegrade the organic compounds. It has been reported that the tall fescue grass (Festuca arundinacea) and switch grass (Panicum virgatum) have the ability to degrade 38% of Pyrene in 190 days [77].
Combined Degradation
The usage of two or more degradation approaches is referred as combined degradation and at present is considered as an approach for the removal of PAHs from contaminated sites. It has advantage over other degradation methods since it is regarded as efficient and cost-effective. Success in combined degradation of PAHs have been documented using pressurize assisted ozonation and integrated treatment using soil washing, ozonation and biological treatment of substrate [78, 79]. Combined degradation and phyto-degradation using inorganic nutrients with E. crassipes is an innovative approach which is gaining a wide acceptability [80].
FACTORS INFLUENCING PAHs DEGRADATION
The biodegradation of hydrocarbons depends on the amount and nature of the hydrocarbon present as well as on the diversity of microbes present. Degradation of PAH depends upon various physical and chemical factors such as soil, pressure, temperature, humidity, nutrient availability, bioavailability and pH. Degradation of PAH insitu by the micro-organisms is highly influenced by temperature. Biodegradation of PAH occurs over a wide range of temperature. At higher temperature bioavailability, solubility and diffusion rate increases which there by enhance the process of biodegradation. However, decrease in oxygen solubility is observed with increase in temperature, thereby reducing metabolic activity of aerobic organisms. Bartha and Bossert [81] and Cooney [82] reported that the maximum degradation occurs at 30-40°C in soil environments, 20-30°C in freshwater and 15-20°C in marine environments. Siron et al. [83], reported the degradation of Naphthalene and Phenanthrene from crude oil in sea water at temperature as low as 0°C. The enzymatic machinery of ligninolytic fungi (Laccase, MnP) having an optimum temperature of 50° C and above 70° C reported over 90% degradation of contaminating PAH in spent-mushroom compost [84]. Degradation of PAH has been recorded in pH range of 2-9. Wong et al. [85], reported degradation of phenanthrene in liquid culture in the range of pH 5.5-7.5 with Burkholderia cocovenenas. Stapleton et al. [86], reported degradation of phenanthrene, naphthalene and anthracene in a soil contaminated with coal spoil at pH 2.0 was evaluated for a period of 28 days. Removal of phenanthrene was 50% and of naphthalene and anthracene was 10% and 20% respectively. Nutrients play a vital role for successful biodegradation of PAHs. For an efficient biodegradation, growth and cellular metabolism ratio of C: N: P is highly critical. However, higher concentration of nutrients can also have an adverse effect on biodegradability. Therefore some nutrients can become a limiting factor affecting the process of degradation. High demands of nutrients by plants make the fresh water wet lands nutrient deficient [87].
Microbial Metabolism of PAHs
Prokaryotes are considered as major decomposers of organic compounds in an ecosystem [88]. Microbes are able to degrade parent compound and substituted PAHs in various environments under aerobic or anaerobic conditions [89, 90]. However, there exist a significant difference in the mechanism of PAH metabolism amongst prokaryote and eukaryotic organisms. Kasnter et al. [91], reported that Gordona, Nocardia, Mycobacterium and Rhodococcus play a key role in degradation of high molecular weight PAH in soil environment. The three main pathways for polycyclic aromatic hydrocarbon degradation by fungi and bacteria are shown below in Fig. (6) [45]. Pointing [92, 93] reported that the member of basidiomycetous group of organisms extensively mineralized the recalcitrant PAHs because of their ability to produce ligninolytic enzymes. Anaerobic metabolism of PAHs is thought to occur via the hydrogenation of the aromatic ring [93].
Bacterial Metabolism of PAHs
Different species of bacteria have been reported for their ability to degrade the PAHs. Bacteria degrade PAH compounds by an assimilative process where they gain carbon and energy for their growth, which leads to mineralization of the compound [48, 94]. The principal mechanism of PAH degradation by bacteria involves its activation and oxidation by enzymes which catalyzes the fixation of oxygen. There are two groups of oxygenases i.e. mono and di oxygenases which incorporate two oxygen atoms into the aromatic ring to form cis-dihydrol. The cis-dihydrol formed are then steroselectively dehydrogenated by cis-dihydrol dehydrogenase which rearomatize the benzene nucleus to form dihydroxylated intermediate (Catechol). Aromatic ring fission of these intermediate occur by the catalytic activity of stero and region selective dioxygenase. The initial ring oxidation is the rate limiting step in the biodegradation of PAH after which the degradation proceeds quickly with very less accumulation of intermediates. The common intermediates produced are catechol, gentisic acids and protocatechuic acid. Thereafter the metabolites are transformed to organic acids and aldehydes [95].
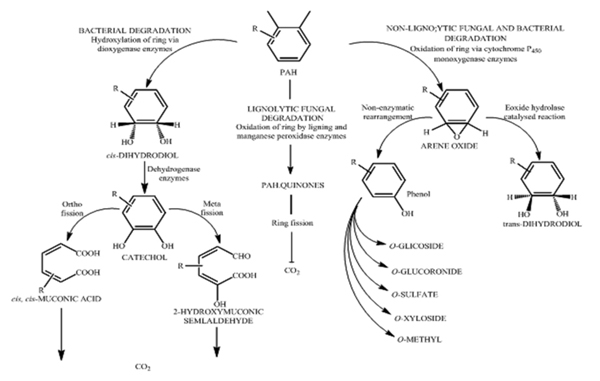
Bacterial catabolism of naphthalene and phenanthrene has been studied extensively. Many bacteria capable of degrading high molecular weight PAH have been reported. Some of the bacterial species involved in degradation of PAHs are as follows: Achromobacter sp. Arthrobacter sp. Bacillus sp. Mycobacterium sp. Burkholderia sp. Pseudomonas sp. Rhodococcus sp. Stenotrophomonas maltophilia, Sphingomonas sp. Xanthamonas sp. Cerniglia [29] suggested that the initial ring oxidation is the rate limiting step in the biodegradation reaction of PAHs. As mentioned in Fig. (7) acetyl CoA and acetaldehyde are the products obtained by ortho and meta pathway respectively. The by-product of these reactions is the production of carbon- di-oxide and water [15]. Gram- negative bacteria, such as Burkholderia (β- proteobacteria) can easily degrade 2 or 3 ring PAHs, where as gram-positive bacteria, such as Mycobacterium, are more efficient in degrading higher ring PAHs [25]. The bacterial consortium (DV-Al) has the ability to degrade naphthalene at a growth rate of 0.0389 g h-1 and at a rate of 80 mg/hr [96]. Wasify and Hamel [97] studied the degradation of crude oil over a period of 28 days and reported its degradation as 88.5% by consortium, 77.8% by Pseudomonas aeruginosa, 76.7% Bacillus subtilis and 74.3% by Acinetobacter lwoffi. PAHs like Fluoranthene and Phenanthrene have been used as a sole source of carbon by Spingomonas sp. [61]. Four or more fused benzene rings PAH (Phenanthrene, Pyrene and Fluoranthene) have been degraded by Mycobacterium. This may be because of the hydrophobic cell surface which helps in adhesion of insoluble PAH thereby facilitating mass transfer of the substrates to cell [30, 98]. The process of degradation of PAH is always affected by environment factors like temperature, moisture and nutrient supply. The isolate Pseudoxanthomonas sp. DMVP2 degrades 300ppm of Phenanthrene within 120 hrs [99].
Fungal Metabolism of PAHs
Like bacteria, fungi also play an important role in bioremediation of PAH in nature. Although the use of PAH as the sole source of carbon by fungi has not been reported extensively. However, several studies have shown that a wide taxonomic and phylogenic spectrum of fungi have the capacity to transform PAH co-metabolically using their enzymatic machinery. A diverse group of lignolytic and non-lignolytic fungi are able to oxidize PAH. Two main groups of enzymes are involved in the initial attack on PAHs by fungi. They are Cytochrome P-450 mono oxygenase and lignin degrading enzyme system. The mechanism of PAH metabolism by non-lignolytic fungi involves the oxidation of aromatic ring by Cytochrome P-450 mono oxygenase [100, 101]. They generally incorporate one atom of oxygen into the aromatic nucleus and reduce the remaining atom to water, followed by enzymatic addition water to yield cis/trans- dihydrodiols [102]. Arene oxide formed can then undergo non-enzymatic rearrangement to form phenol which can further be conjugated with glucose, xylose, gluconeric acid and sulfate. Various non-lignolytic fungi like A. niger, C. elegans, P. janthinellum used Cytochrome P 450 monoxygenase for the oxidative degradation of PAH. The most widely studied non-lignolytic fungus is C. elegans. An example of Cytochrome P450 - mediated oxidation of Phenanthrene is shown in Fig. (8)[98].
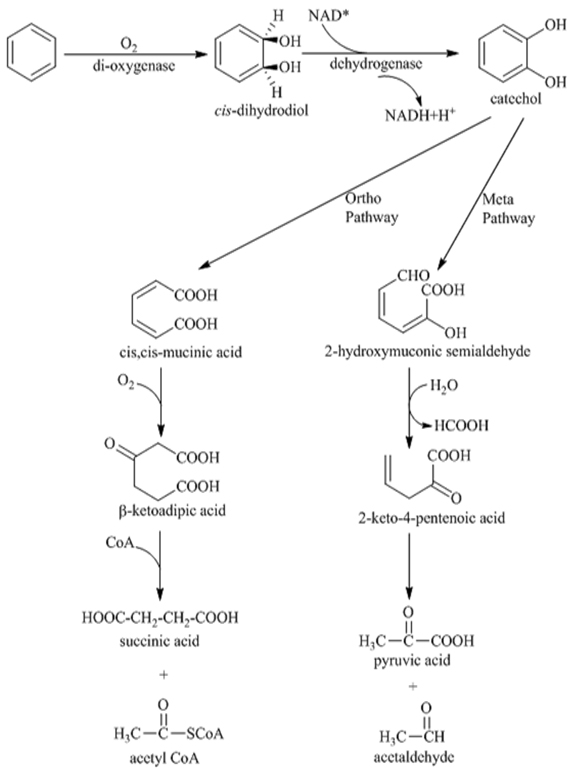
Ligninolytic Fungi
Ligninolytic fungi commonly known as white rot fungi as it causes white rot of woods. White rot fungi have been screened for PAH degrading abilities when grown under ligninolytic and non-ligninolytic culture condition. The generas of Phanerochaete, Trametes, Bjerkandera and Pleurotus have shown considerable potential for the degradation of PAH [103, 104]. The white rot fungi produce extracellular lignin degrading enzyme which have the potential to degrade xenobiotic compounds. Fungal extracellular enzymes responsible for the degradation of lignin, LiP, MnP and laccase are believed to be involoved in the degradation of PAHs [105, 106]. Ligninolytic fungi find wide spread application in biodegradation of PAH to CO2 and O2. Phanerochaete chrysosporium has been generally used as model organism to study potential for degradation of PAH. Initial attack of Phanerochaete chrysosporium under culture condition that favour lignolysis is catalysed by enzymes like LiP and MnP. Some of the white rot fungi metabolize PAH to their quinones and other metabolites that do not appear to involve a cis-trans dihydrodiol [107]. White rot fungi has the ability to produce metabolites with a higher water solubility and chemical reactivity than the parent PAH. Mono or dihydroxylated PAH metabolites could be substrates for bacteria and their degradation a reaction in soil is caused by indigenous micro flora [30]. Manganese peroxidase is thought to work through a similar mechanism of electron oxidation with diffusible oxidizing agent Mn+3- chelate, which is a weak oxidant [108]. It is incapable of oxidizing PAHs with ionization potentials equal to or greater than chrysene (5-7.8eV). The system mediated by the peroxidation of unsaturated lipids by MnP and Mn+3 was reported to catalyze the oxidative cleavage of phenanthrene to diphenic acid [109]. Application of laccase in PAH degradation has been well studied. Laccase catalyzes one electron oxidation of PAHs such as Anthracene and Benzo (a) Pyrene both having ionization potentials 57.55eV. Collins et al. [110], reported degradation of anthracene and Benzo (a) Pyrene using Trametes versicolor by crude as well as two purified isoenzymes . Level of oxidation of athracene was higher by two purified laccase with ABTS [110]. Phenanthrene is oxidized initially to Phenanthrene- 9, 10-quinone and thereafter by the process of ring cleavage to 2.2’-diphenic acid by Phanerochaete chrysosporium [111, 112]. as shown in Fig. (8). It was observed that maximum degradation of 84.98% was obtained on the 15th day of incubation by Agaricomycetes sp AGAT [113]. Sack et al. [114], reported 13.8% of degradation of pyrene after 63 days of incubation by Trametes versicolor. Lea et al. [115], reported that when Pleurotus ostreatus was grown in the presence of [14C] Pyrene, 91% pyrene was metabolized. Hadibarata and Teh [116] reported that maximum degradation rate (99%) was obtained at 10 ppm concentration after incubation of 30 days.
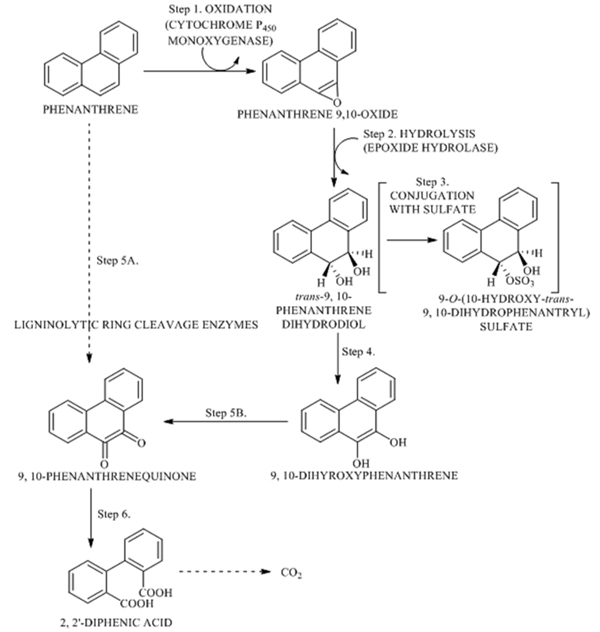
PAH are formed during the incomplete combustion of organic material and in connection with the worldwide use of oil, gas, coal and wood in energy production. The wide spread sources of PAHs present everywhere, make them a complex mixtures of chemicals, including derivates of PAH. Exposure to PAHs or their derivatives at workplace or in house have an adverse effect on the human body. Therefore health risk evaluation is necessary.
Various government agencies worldwide have established stringent norms relating to the PAH in air, soil and water. OSHA in USA has established the permissible PAH limit to 0.2 mg/ m3 for 8 hour TWA (Time-weighed average) [117]. NIOSH in USA has recommended the limit of 0.1 mg/m3 for coal tar pitch [118]. EPA has suggested that the intake of individual PAH which is not likely to be deleterious on human body at the level is: Anthracene (0.3 mg), Phenathrene (0.04 mg) and Pyrene (0.03 mg) per kg of body weight. Total exposure of PAH should not exceed 3 mg/ day [119]. EPA has also decided the concentration of Benzo (a) pyrene not to exceed 0.2 ppb in water as it is considered to be the most carcinogenic PAH [120].
CONCLUSION
Polycyclic aromatic hydrocarbons (PAHs) are the set of compounds known as polycyclic organic matters (POM). They are an important part of environment contaminants which are originally produced from incomplete combustion of organic materials or pyrolysis of organic materials. The fate of PAH in nature is of great environmental concern due to its mutagenic and carcinogenic toxicity. Biodegradation of PAHs and other xenobiotic compounds depend upon micro organisms to either transform or mineralize them to CO2 and H2O. The present study notes that the effective depolluting of PAH from contaminated site with the help of physical, chemical, combined bioremediation and phytoremediation approaches appear to be most efficient and cost effective environmental friendly to decontaminate PAH. The development and use of genetically modified organisms represent the research friendlier environment. However, the release of such organism is always questionable. Therefore, based on the present review it may be concluded that the use of microbes is a green degradation method for the cleanup of the PAH contaminated sites.
CONFLICT OF INTEREST
The authors confirm that this article content has no conflict of interest.
ACKNOWLEDGEMENTS
The authors are grateful to Department of Biotechnology (BT/PR7567/BCE/8/1005/2013), Ministry Of Science and Technology Govt. of India, New Delhi for their financial support and Sophisticated Instrumentation Centre for Applied Research and Testing (SICART) for providing instrumentation facility for the successful completion of this work.