All published articles of this journal are available on ScienceDirect.
Preparation and Characterization of Large Unilamellar Vesicles Mixed With Trimethylchitosan (TMC): The Effect of Polyelectrolyte Concentration
Abstract
Background:
The effect of different concentrations of the absorption enhancer Trimethyl Chitosan (TMC) to the physicochemical properties of Large Unilamellar Vesicles (LUV) comprised of L-a-Phospahtidyl Choline (PC) were investigated in the current study.
Methods:
The Degree of Quartenization (DQ) of trimethylchitosan was assessed with nuclear magnetic resonance (1H NMR). The vesicles were characterized by means of Dynamic Light Scattering (DLS), ζ-potential, Differential Scanning Calorimetry (DSC) and Contact Angle Goniometry (CAG) measurements.
Results:
The data showed that the surface charge of the PC liposomes was significantly altered as a function of the TMC concentration, giving evidence of presence of the polyelectrolyte to the liposome’s membrane. Varying the concentration of TMC affected the phase Transition Temperature (Tm) of the lipid, verifying the miscibility of the polyelectrolyte with the lipid bilayer. The association of the polymer with the liposomes was related to the amount of the polyelectrolyte present, reflecting changes to the wettability of the dispersion as measured by CAG.
Conclusion:
The results demonstrated that presence of TMC significantly modified the physical properties of liposomes. Such systems might have a potential use for mucosal delivery (e.g. nasal route of administration).
1. INTRODUCTION
The use of colloidal drug delivery systems, such as nanoparticles and liposomes, has been shown to increase the bioavailability of administered drugs (REF). Liposomes, which are vesicles, composed of one or more phospholipid bilayers can carry a variety of molecules with therapeutic potential and offer different advantages for many routes of administration [1]. Furthermore, liposomes decorated with polyelectrolytes, alter their properties, are extensively used as drug carriers [2, 3] and have been shown to increase their therapeutic activity and circulation lifetime in a biological milieu [4]. By combining chitosan and liposomal characteristics, specific, prolonged, and controlled release can be achieved [5-7]. A solid polymeric vesicle, namely palmitoyl glycol chitosan, was entrapped within a liposomal formulation to control the release profiles of a model compound [8]. A small degree of mixing of the lipids occurred upon hydration of the lipid/surfactant film, resulting in a vesicle formulation instead of a vesicle in vesicle system [8]. The results showed that the polymeric vesicle phospholipid in vesicle system released 28% of the entrapped model compound compared to 62% from the polymeric vesicles over the duration of 4 hours [8].
The presence of chitosan can also enhance the mucoadhesive properties of liposomes. The extent of in vitro mucoadhesion of liposomes coated with different polymers, including chitosan, and carbopol, has been reported previously [9]. The results suggested that chitosan-coated liposomes had the highest percentage of mucoadhesion, followed by carbopol, where no adhesive percentage was observed for the non-coated liposomes [8]. This indicates that the polymer physically interpenetrates the mucin layer in order to facilitate liposome adherence. In a previous report the effect of concentration and different molecular weight of TMC to liposomes containing Coenzyme Q10 was investigated [10]. In the recent studies TMC derivatives co-formulated with liposomes were utilized for ocular deliver of vitamin A palmitate [11], nasal delivery of BSA [12], oral absorption of calcitonin and curcumin [13, 14]. The existence of a polymer layer on the surface of the formulation was confirmed by means of electron microscopy and was associated with changes in particle size diameter and zeta (ζ) potential values. In the current study, Large Unilamellar Vesicles (LUV) with variable TMC (N-trimethyl chitosan) concentration were prepared by the thin-film hydration method and the resulting colloidal dispersions were characterized by means of Dynamic Light Scattering (DLS), ζ-potential measurements, Differential Scanning Calorimetry (DSC), and Contact Angle Goniometry studies (CAG).
2. MATERIALS AND METHODS
2.1. TMC Synthesis
TMC was synthesized from high viscosity chitosan as described previously [11]. 1H-NMR was used to characterize the synthesized TMC polymer. The 1H-NMR spectrum was recorded in D2O with a Bruker 600 MHz spectrometer (Bruker, Faellanden, Switzerland) at 80°C. Minimum interference was observed from the water peak at this temperature and suppression of the water peak was not necessary.
2.2. Preparation and Characterization of TMC/LUV Liposomes
Several approaches have been employed for the formation large vesicles. In a recent study large vesicles were formed combining an electric field and calcium ions [15]. Large Unilamellar Vesicles (LUV), containing TMC, consisted from L-a-Phosphatidyl Choline (PC) (Avanti Lipids, Alabama US) were prepared as has been previously described [16]. Briefly, 13 μmol of the lipid (PC) was dissolved in chloroform in a spherical flask. The organic solvent was removed in a rotary evaporator and the thin film was rehydrated with 2 mL of PBS pH 7.4 containing different amounts of TMC (0, 0.25, 0.50 and 1% w/v respectively). Highly purified water (18.2 MΩ.cm) was used for the preparation of all the solutions. The dispersion was subjected to temperature controlled (20 oC) bath sonication for 20 minutes. The LUV were prepared by extrusion through two stacked polycarbonate filters of 0.4 μm pore size (Nucleopore, Corning Costar Corp., MA, USA) under nitrogen pressure 30 psi.
2.3. Dynamic Light Scattering (DLS) and ζ-Potential Studies
The size distribution (mean diameter and polydispersity index) and the zeta (ζ) potential of the liposomes were measured by DLS on a ZetasizerNano-ZS (Malvern Instruments Ltd., UK), which enabled to obtain the mass distribution of particle size as well as the electrophoretic mobility. Measurements were made at 25 °C with a fixed angle of 137 °. Samples for three different batches were measured.
2.4. Differential Scanning Calorimetry (DSC) Studies
Thermal analysis of the formulations was performed using a Perkin Elmer calorimeter (Perkin Elmer Diamond DSC) in a nitrogen atmosphere. Samples consisted of a 30 μL of liposome suspension in PBS buffer were placed in standard aluminium DSC pans of 40 μL with a pierced lid at a heating scan rate of 2 oC/min.
2.5. Contact Angle Goniometry (CAG) Studies
Advancing (θA) contact angles of small drops (2 μL) at 20 °C were measured using a goniometer with an enclosed thermostated cell (Kruss G10, Hamburg, Germany). The contact angles of droplets (× 3) placed on the horizontal PMMA (× 2) and were obtained for both ‘left’ and ‘right’ contact after approximately 15 s after the initial drop-surface contact. PMMA was used as a model substrate because its surface free energy is a reasonable estimate for hydrophobic compounds which are known to have problematic wetting.
3. RESULTS AND DISCUSSION
3.1. Characterization of TMC
A yield of 67% was obtained with the synthesis). The polymer synthesised was characterised by 1H-NMR to determine the degree of quarternization, which indicates the percentage of N-groups methylated to quaternary ammonium groups. The degree of substitution was 21.2% which falls within the range typically observed for a one step synthesis reported previously [17].
3.2. Size Distribution and ζ-Potential Data
The effect of different amounts of TMC on the particle size of PC liposomes is illustrated in Fig. (1A). The particle size of TMC-PC liposomes is decreased with increasing concentration of polymer solution. More specifically, the particle size of plain liposomes decreased from 350 to 150 nm with the addition of 0.25% w/v TMC, followed by a decrease to 115 nm and 150 nm when 0.5% and 1% w/v of TMC were added, respectively. The Polydispersity Index (PDI) values for 0, 0.25%, 0.5% and 1% were respectively 0.157, 0.198, 0.245 and 0.259, respectively. This is suggestive of interaction between the polymer and the lipid bilayer (Fig. 1A). The electrical properties of the liposomes in the presence of TMC were assessed by means of ζ-potential measurements. The ζ-potential values for both formulations are shown in (Fig. 2B). PC is a zwitterionic lipid with no net charge [18] while TMC at pH 7.4 is positively charged due to the quaternary ammonium groups. An increase to the ζ-potential values of the PC formulation from -0,65 mV (plain liposomes) to 8.46 mV (1% w/v TMC) was observed after increasing the amount of polyelectrolyte, indicating the incorporation of the polymer in the vesicles’ structure, possible as a layer on the surface of the vesicles.
The relative low values of ζ-potential measured for the liposomal formulations could be attributed to the high ionic strength of the buffer (PBS, pH 7.4) which suppresses the thickness of the electric double layer of the vesicles [18].
3.3. DSC and CAG Studies
To further investigate the interactions of the produced vesicles DSC and contact angle goniometry were employed. The miscibility of TMC with PC liposomes was assessed by means of DSC. Plain PC liposomes have a clear endotherm transition peak at Tm = -10,6°C (Fig. 2A), which is in line with values reported previously [19]. This suggests that the presence of TMC induced changes to the main Transition Temperature, Tm, of the liposomes. More specifically, when 0.25% w/v of TMC was added to vesicles, the Tm was dramatically decreased from -10.8°C to -18.5°C, and was further decreased to -19.3°C and -18.9°C when 0.5% w/v and 1% w/v of TMC was respectively present in the membrane an indication of the miscibility of polyelectrolyte with the phospholipid (Fig. 2A). The main transition is shifted by the presence of TMC in both formulations due to a change, from a gel-like to a liquid-like packing, of the hydrophobic tails of the lipids [20]. That suggests that the packing of the lipids is disturbed within the bilayer; i.e. their order is reduced. This can be attributed to the penetration of the polyelectrolyte into the lipid bilayers. The changes noticed with PC liposomes advocating that the length of the fatty acyl chain seems to play a role on the localization of the polyelectrolyte into the membrane. In an attempt to investigate the potential changes of the lipophilic character of the liposomes upon the addition of the polyelectrolyte, Contact Angle Goniometry (CAG) studies were performed. Fig. (2B) illustrates the CAG values obtained for TMC-PC and liposomes as a function of the polyelectrolyte concentration. In the PC formulation, the θ° value of plain liposomes is increased significantly from 63.42 to 72 (t-test p<0.05) when 0.25% of the polyelectrolyte was added to the vesicles indicating that TMC is located in the lipid bilayer. However, the contact angle values declined up to 55.75 after the addition of high concentrations of TMC (1% w/v). The significantly lower CAG values, compared with the control (t-test p<0.001), confirm the presence of hydrophilic moieties of the polyelectrolyte (TMC) to the surface of liposomes.
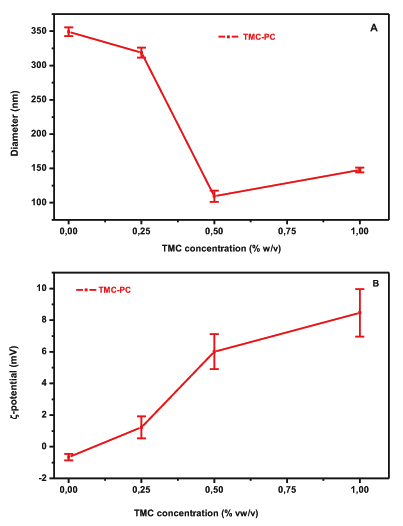
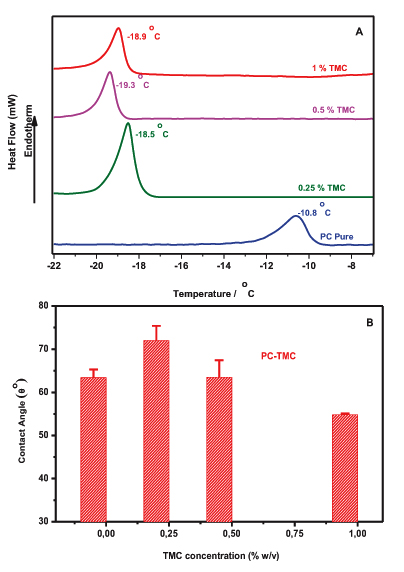
CONCLUSION
The current results emphasize that the amount of the polyelectrolyte that is present in the liposomes’ structure can affect the physicochemical properties of the resulted vesicles. TMC has extensively been used as an absorption enhancer for peptide and protein drugs and possesses mucoadhesive properties [21]. Mixed liposomes with TMC might be a beneficial approach for mucosal delivery (e.g. nasal administration).
ETHICS APPROVAL AND CONSENT TO PARTICIPATE
Not applicable.
HUMAN AND ANIMAL RIGHTS
No Animals/Humans were used for studies that are base of this research.
CONSENT FOR PUBLICATION
Not applicable.
CONFLICT OF INTEREST
The authors declare no conflict of interest, financial or otherwise.
ACKNOWLEDGEMENTS
Declared none.