All published articles of this journal are available on ScienceDirect.
Epigenetic Reprogramming in the Mammalian Germ Line: Possible Effects by Endocrine Disruptors on Primordial Germ Cells
Abstract
The present work provides a brief review about evidence obtained in the past years mainly in our laboratory using the mouse model, that germ cells and gonadal somatic cells may be direct target of endocrine disruptors (EDs) from very early stages of gonad formation. Since it is now known that epigenetic pathways are crucial for germline development and that EDs are also able to interfere with epigenetic mechanisms, we will discuss these results mostly in light of possible effects by such molecules on the epigenoma of the primordial germ cells (PGCs), the precursors of the adult gametes that transmit genetic information between generations.
INTRODUCTION
On the basis of endogenous and exogenous environmental signals, epigenetic mechanisms of gene regulation involving DNA methylation, histone modifications, changes of chromatin structure and microRNA expression, organize the genome into active and inactive domains, representing crucial players of gene expression. Key epigenetic modifiers of the genomic DNA are the methyltransferases (DNMTs), the methyl-CpG (MeC)-binding proteins and the histone-modifying enzymes. Among these, DNMT1 is a maintenance DNA methyltransferase whereas DNMT3A and DNMT3B function in de novo methylation. Epigenetic regulation of genes encoding these enzymes, the MeC-binding proteins or the microRNA expression by hormones provides ways of interplay between the endocrine system and epigenetics. These interactions can be insidiously modulated by a class of compounds termed as endocrine disruptors (EDs). EDs are environmental chemicals that mimic hormones or exert anti-hormone activities, and alter the physiologic function of endogenous hormones. Plants are the sources of some of these chemicals, named phytoestrogens, while others are natural substances such as heavy metals or synthetic molecules or drugs. Several reports indicate that in mammals and a number of other species, EDs may be detrimental to reproduction by promoting abnormalities in sex differentiation and gonad functions, including testicular cancer in the male and ovarian diseases in the female (for a review, see [1]). However, the exact mechanisms of the ED action on the reproductive system are not completely known.
In the present work, we briefly review evidence coming mostly from results obtained in our laboratory in the mouse, that germ cells and gonadal somatic cells may be direct target of EDs from very early stages of gonad formation during the embryonic life. Since it is now emerging that epigenetic pathways are crucial for germline development and that EDs are able to interfere with epigenetic mechanisms, we will discuss these results in light of possible effects by such molecules on the epigenoma of the primordial germ cells (PGCs), the precursors of the adult gametes. PGCs appear at early stages of embryonic development and differentiate into oogonia/oocytes or prospermatogonia/gonocytes in the female and male foetal gonads, respectively. After birth, oocytes and gonocytes finally give rise to haploid oocytes and sperm that upon fertilization produces new individuals. In principle, alteration of epigenetic dynamics in PGCs may lead not only to defects in germ cell development and adult fertility but it might be transmitted to the next generation with possible onset of diseases in the adults.
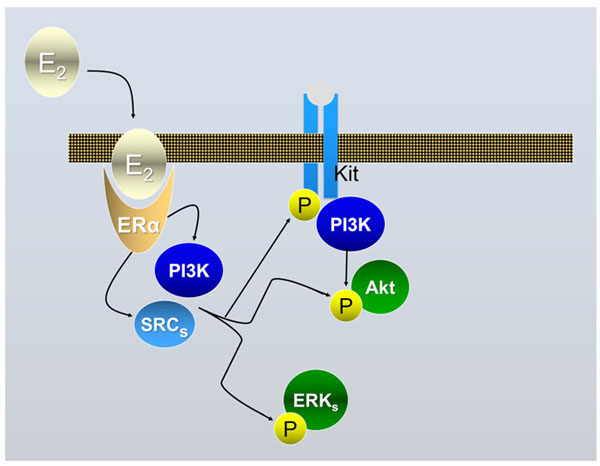
Schematic drawing of the ERα-dependent E2/ED activable pathways in mouse PGCs following the results described in [2]. Binding of E2 or EDs to ERα allows the formation of a complex with MNAR that binds SRCs and leads to its activation; the complex binds and phosphorylates (activates) PI3K and SRC. Upon activation PI3K mediates AKT phosphorylation (activation); SRC via RAS and RAF are also able to trigger the activation pathway of ERKs (mainly ERK2). Eventually, SRC are responsible to cross-phosphorylation of the KIT receptor through an unknown pathway; this eventually also leads to the activation of the PI3K/AKT pathway.
ENDOCRINE DISRUPTORS AND REPRODUCTIVE TOXICOLOGY
There is little doubt that exposure to EDs may promote abnormalities in the reproductive system. For example, in many species EDs alter sex differentiation and are regarded responsible for the decrease in sperm count and an increase in testicular cancer incidence in humans. Examples of the environmental EDs postulated to have adverse effects on the reproductive system in animals including humans are pesticides (e.g., methoxychlor), fungicides (e.g., vinclozolin), a range of xenoestrogens (EDs with estrogenic activity), like bisphenol A (BPA) and certain phthalates (for a review, see, [1]). Environmental xenoestrogens are likely to elicit their actions through the two canonical mammalian receptors for estrogens (ERα and ERβ), widely expressed in the gonads and the reproductive tracts. Several studies reported the expression of ERα and ERβ in rodent and human prenatal and postnatal ovaries and testes both by germ cells and somatic cells ([2] and references here in). Relevant for the present review, we found that ERα is expressed both by PGCs [2] and somatic cells [3] of mouse sex indifferent gonads. Moreover, results obtained in our and other laboratories showed that the development of mammalian PGCs and spermatogonia (namely their proliferation/survival), could be modulated and/or altered by estrogens and some EDs both directly or indirectly through neighbouring somatic cells , as the results of genomic and nongenomic effects ([2, 3] and references here in). In some cases, the molecular mechanisms underlying the ED effect on germ cells have been identified. For example, we found that 17-β-estradiol (E2) was able to rapidly stimulate AKT kinase, KIT receptor, ERK2 and SRC kinase phosphorylation in mouse PGCs and to promote their survival/proliferation [2] (Fig. 1). On the other hand, the level of AKT activity significantly decreased in mouse PGCs exposed to lindane (γ-HCH) in vitro along with the increase in the number of apoptotic germ cells either in culture and in the embryo [4]. Likewise, others found that a G-protein-coupled receptor-30 (GPR-30) mediates E2-induced proliferation of chicken PGCs through EGFR/AKT/β-catenin signaling pathway [5]. Other studies showed various responses by mouse PGCs to EDs likely through different mechanisms of action [6]. For example, in vitro cultured PGCs exposed to mono (2-ethylhexyl) phthalate (MEHP) (the direct metabolite of the 2-ethyl-hexyl-phthalate or DEHP), a widespread plasticizer, affected PGC adhesion to cell monolayers likely causing alteration of germ cell-somatic cell interactions crucial for gonad development [7]. In order to identify gene deregulated by different EDs and reprotoxicants, cDNA libraries prepared from small number of mouse PGCs followed by differential screening, showed altered gene expression in these cells after in vitro exposure to N-ethyl-N-nitrosurea (ENU), adriamycin and MEPH [1]. Most of deregulated genes detected encoded proteins involved in cell survival pathways such as respiratory chain and oxidative stress, ribosomal proteins, metabolism of the cell and translation factors. Other studies carried out in vitro on somatic cells of mouse embryonic testes, likely pre-Leydig cells, showed that E2 and lindane were able to activate specific DNA estrogenic responsive elements (ERE) [8] and caused deregulation of cell cycle genes in such cells [9] such as Cdk1c (Cyclin-dependent kinase inhibitor 1C or p57 or Kip2), Ccd3 (Cyclin D3) and Rbl1 (Retinoblastoma-like 1) (Fig. 2). All together these results, showed for the first time that both PGCs and somatic cells of the embryonic gonad possess functional estrogenic receptors able to activate genomic and nongenomic pathways and therefore potential targets for estrogenic ED compounds.
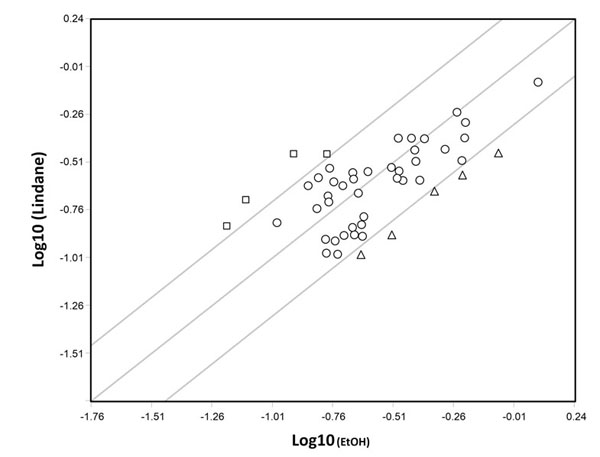
Scatter plot analysis representing the comparison of the log10-scaled expression signal of the transcripts of 40 cell-cycle-related genes showing significative expression in somatic cells obtained from E12.5 mouse testis and cultured for 24 hr in the presence of 10-5 M lindane and vehicle EtOH. Circles represent transcripts whose expression were not affected by lindane while triangles and squares represent genes downregulated (Abl1, Apbb1, Ccnd3, Cdkn1c, Dst) and upregulated (Mcm7, Rad9, Ran, Rbl1), respectively (> 2-fold change). Unpublished results (for details see [9]).
GERM-LINE DEVELOPMENT AND EPIGENETIC
Germ cells are unique among the various cell types since they give rise to a new organism and transmit genetic information to the next generations. Therefore, in these cells, epigenetics plays a basically different role than in somatic cells. In fact, while in these latter epigenetic changes make gene-expression programs progressively more restricted through successive differentiation pathways, in germ cells the genome retain intrinsic totipotency. The main aspects of germ cell development that are linked to epigenetic events are thought to be mainly the need for a unique gene-expression program that maintains such intrinsic totipotency and at the same time allows a well-defined differentiation, the fact that germ cells undergo meiosis and the particular importance of maintaining genomic integrity in these cells. Although epigenetic modifications occur in germ cells throughout both male and female gametogenesis, those occurring during PGC development have been the object of the most recent and intense studies because they are not only crucial for germline segregation but offer several clues to understand some secrets of stem cell biology. Several reviews provide the reader with a compressive overview of these processes [10-14], and therefore will be only briefly reported here. While studies carried out in the mouse embryo, indicate that the bulk of the genome-wide epigenetic reprogramming in PGCs occurs after the colonization of the gonadal ridges, at least a part of the reprogramming process starts much earlier, nearly concomitantly with PGC specification, and proceeds during the PGC migration period. By the time of gonad sex differentiation, genome-wide DNA demethylation in PGCs leads to the erasure of genomic imprinting, partly demethylation of the transposable/repetitive elements and reactivation of the inactivated X chromosome in females. At the same time, the PGC genoma appear to undergo a number of histone modifications and a global reorganization of the chromatin structure. Once the parental imprints have been erased, new imprints are re-established according to the sex of the animal. Such imprint occurs after sex determination that has been initiated, and male and female germ-cell development diverges to give rise to sperm or eggs. In male, paternal methylation imprints are progressively established in germ cells between the end of the fetal and the newborn stages. In the female germline, the initiation of DNA methylation imprinting occurs after birth, during the oocyte growth. The growing oocytes are blocked at the diplotene stage of meiotic prophase I, and the de novo methylation process is complete by the fully-grown oocyte stage. Besides DNA demethylation and methylation, several changes in histone occur during meiosis and the final stages of both female and male gametogenesis that complete the dynamics of the germ cell epigenetics.
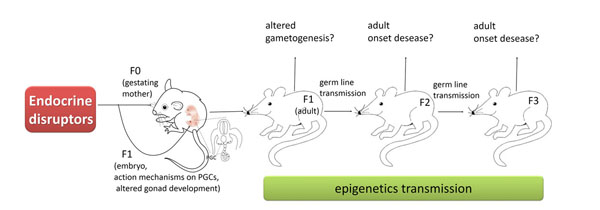
Role of the PGCs in epigenetic transgenerational inheritance. EDs acting on the F0 generation gestating female influence the developing F1 generation foetus and alter gonadal development to reprogram the PGC DNA methylation. This epigenetic alteration in the germline may become permanent and is transferred through the germline to subsequent generations. The embryo generated from this germline posses an altered epigenome that affects developing somatic cells and tissues. This altered somatic cell transcriptome can then promote adult-onset disease associated with the transgenerational phenotype.
Given the evidence that EDs can affect epigenetics, for example by modifying the DNA methylation status and/or inhibiting histone deacetylase activity (for a review see [15]) and, as reported above, the presence functional estrogenic receptors in PGCs and the companion somatic cells, it is reasonable to think that EDs, at least those with estrogenic activity, might interfere with the germ cell reprogramming through epigenetic mechanisms. These effects can result in immediate abnormalities in germ cell development and/or cause transgenerationaleffects on next generations. This provides a unique epigenetic mechanism to promote a transgenerationalphenotype induced by an environmental factor including, besides testis and/or ovary abnormalities, tumours and pathological development in a variety of somatic tissues [15].
Actually, there has always been much interest in the idea that some epigenetic marks can be inherited across generations. However, despite the fact that these marks are considered relatively stable during development, they undergo resetting in PGCs and subsequently in the zygote at fertilization to ensure the totipotency of cells of the early embryo. For transgenerational epigenetic inheritance to occur this reprogramming must be, however, bypassed (Fig. 3). Recent results indicate that some sites of the genome can, actually, evade erasure of DNA methylation occurring in PGCs [16-18]. But evidence that EDs can impose transmissible epigenetic mark on PGCs is hotly debated.
Actually, some papers from the Skinner‘group support such a possibility. These authors reported that maternal exposure to certain environmental conditions and in particular to some EDs, result in transgenerational phenotype throughout epigenetic alterations in the germ-line. In particular, they showed that transient male rat embryo exposure to vinclozolin at the time of PGC development remarkably caused a transgenerational phenotype in F1-F4 generations of male germ cell apoptosis and subfertility. This apparent epigenetic mechanism involves altered DNA methylation and permanent re-programming of the male germline. In fact, a series of genes with altered DNA methylation and imprinting were identified in PGCs and later in sperm [19]. The rat model was also used to evaluate whether adult onset ovarian diseases could be induced transgenerationally after exposure of a gestating F0 generation female to known environmental toxicants such as vinclozolin and a mixture of polycarbonate plastics such as BPA, dibulylphthalate (DBP) and bis(2-ethylexyl) phthalate (DEHP), during the period of PGC migration and gonadal ridges formation [20]. The results showed that the environmental toxicants examined induced transgenerational ovarian adult-onset disease resembling human ovarian insufficiency (POI) and polycystic ovarian syndrome (PCOS), thus suggesting that such ovarian disease can have an epigenetic transgenerational etiology. Despite such results, others found that undernourishment during the prenatal life may compromise F1 sperm methylation but such change is not transmitted to F2 offspring; nonetheless, gene expression is altered in somatic cells of these F2 offspring at regions of F1 germline differential methylation [21], leaving open the way to alternative possibilities for epigenetic trans-genarational transmission.
CONCLUSION
The epigenetic germ-line transgenerational disease hypothesis provides a unique perspective from which to view adult onset disease caused by endocrine disruptors and ultimately offers new insights into novel prevention and diagnostic and therapeutic approaches. Moreover, the researches in this field contribute to our understanding of the epigeneticmechanisms underlying imprinting erase and acquisition during germcell development and may have implications for assistedreproductive technologies. With the rapid advances in technologies for high-throughput global screening of DNA methylation, histone modifications, and small RNA profiling and the advent of next-generation sequencing technologies, we expect an explosive phase of growth in genome and epigenome science. Further studies on PGCs should focus on the molecular pathways activated by EDs leading to alteration of DNA methylation or other epigenetic modifiers. Along with these advances will be significant for our understanding of the interplay of epigenetics and genetics with environment compounds in modulating the endocrine system at the individual and population level and of the etiology of several diseases.
CONFLICT OF INTEREST
The authors confirm that this article content has no conflict of interest.
ACKNOWLEDGEMENTS
Declared none.